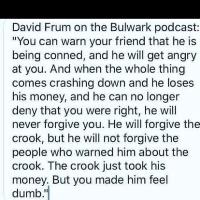
Thursday, September 30, 2021 6:49:13 AM
Here's the excerpt that contains the only mention of brilacidin.
"As of now, there are two exceptional drugs that can work on any new strain of bacterium. One is ACHN-975, and the other is Brilacidin. The former is a first-line emergency medication as an initial defense against most infectious species. The latter is still in clinical trials. The mode of action is seen to be LpxC inhibition. This enzyme is responsible for lipid A synthesis in pathogens."
As KMBJN remarked this claim is underdetermined by actual data to the point of absurdity.
Full text:
Role of nanomaterials in deactivating multiple drug resistance efflux pumps
– A review
Nibedita Dey a, C. Kamatchi b, A.S. Vickram a, K. Anbarasu c, S. Thanigaivel d, Jeyanthi Palanivelu e, Arivalagan Pugazhendhi f, Vinoth Kumar Ponnusamy g, h, i,*
a Department of Biotechnology, Saveetha School of Engineering, Saveetha Institute of Medical and Technical Sciences, Chennai, India
b Department of Biotechnology, The Oxford College of Science, Bengaluru, India
c Department of Bioinformatics, Saveetha School of Engineering, Saveetha Institute of Medical and Technical Sciences, Chennai, India
d Department of Biomedical Engineering, Saveetha School of Engineering, Saveetha Institute of Medical and Technical Sciences, Chennai, India
e Department of Biotechnology, Vel Tech Rangarajan Dr. Sagunthala R&D Institute of Science and Technology, Chennai, India
f School of Renewable Energy, Maejo University, Chiang Mai, 50290, Thailand
g Department of Medicinal and Applied Chemistry & Research Center for Environmental Medicine, Kaohsiung Medical University (KMU), Kaohsiung City, 807, Taiwan
h Department of Medical Research, Kaohsiung Medical University Hospital (KMUH), Kaohsiung City, 807, Taiwan
i Program of Aquatic Science and Technology, College of Hydrosphere Science, National Kaohsiung University of Science and Technology (NKUST), Kaohsiung City, Taiwan
A R T I C L E I N F O
Keywords:
Antibacterial properties Drug resistance
Efflux system Nanoparticles Nano therapy Synergistic effect
Enjoy:
A B S T R A C T
The changes in lifestyle and living conditions have affected not only humans but also microorganisms. As man invents new drugs and therapies, pathogens alter themselves to survive and thrive. Multiple drug resistance (MDR) is the talk of the town for decades now. Many generations of medications have been termed useless as MDR rises among the infectious population. The surge in nanotechnology has brought a new hope in reducing this aspect of resistance in pathogens. It has been observed in several laboratory-based studies that the use of nanoparticles had a synergistic effect on the antibiotic being administered to the pathogen; several resistant strains scummed to the stress created by the nanoparticles and became susceptible to the drug. The major cause of resistance to date is the efflux system, which makes the latest generation of antibiotics ineffective without reaching the target site. If species-specific nanomaterials are used to control the activity of efflux pumps, it could revolutionize the field of medicine and make the previous generation resistant medications active once again. Therefore, the current study was devised to assess and review nanoparticles’ role on efflux systems and discuss how specialized particles can be designed towards an infectious host’s particular drug ejection systems.
1. Introduction
Nanotechnology has found its way into almost all research fields due to its particular dimension, and surface-to-volume ratios harbor unique features of great potentials (Prabhu and Poulose, 2012b). Nanotech- nology has been employed to control bacterial growth and resistance to a great extent in microbiology (Chatterjee et al., 2014). The growing crisis of increasing resistance to various antibiotics is a significant concern that the world faces today (Ayukekbong et al., 2017). Multidrug-resistant organisms (MDROs) are the most life-threatening (Boucher et al., 2009). They are the prime reason behind morbidity and fatality in all age groups (Ismail et al., 2018). For the formulation
and development of recent generation antibiotics, enormous amounts of labor and funding are required (Spengler et al., 2017). Table 1 depicts the dosage-based resistance pattern in pathogens (Badar and Khan, 2020). Even bringing a new drug into the market after all the trials is time-consuming. As pathogens are exposed to increasing concentrations of drugs, they tend to become tolerant to the specific drug (Hassan et al., 2015a). Their cellular mechanism is equipped to find alternate strategies to make these static and cidal drugs as tolerable toxins (Ramirez et al., 2013). Hence, it’s always appreciated to find ways to avoid the tendency of the organism to gain resistance. Table 2 represents the average rate of resistance prevalent among World Health Organization (WHO) priority pathogens towards the corresponding antibiotics in India. There has
* Corresponding author. Department of Medicinal and Applied Chemistry & Research Center for Environmental Medicine, Kaohsiung Medical University (KMU), Kaohsiung City, 807, Taiwan.
E-mail addresses: pugal.smile@gmail.com (A. Pugazhendhi), kumar@kmu.edu.tw (V.K. Ponnusamy).
https://doi.org/10.1016/j.envres.2021.111968
Received 17 May 2021; Received in revised form 5 August 2021; Accepted 19 August 2021
Available online 26 August 2021
0013-9351/© 2021 Elsevier Inc. All rights reserved.
Table 1
Comprehensive dosage-based resistance pattern in pathogens (Badar and Khan, 2020).
Medication Dosage (mg) Organisms
Ampicillin 10 K. pneumoniae, S. epidermidis, S. maltophilia
Amikacin A. baumanii
Cefepime 30 S. epidermidis, Enterobacteriaceae
Cefotaxime 30 S. epidermidis, E. coli
Ceftazidime 30 S. epidermidis
Cefuroxime 30 K. pneumoniae, S. epidermidis
Ciprofloxacin 5 K. pneumoniae, A. baumanii
Gentamicin 10 K. pneumoniae, A. baumanii, S. maltophilia
Imipenem 10 A. baumanii, S. maltophilia
Meropenem 10 A. baumanii, S. maltophilia
Methicillin – S. aureus
Trimethoprim 5 S. maltophilia
Levofloxacin 5 A. baumanii
Table 2
Average rate of resistance prevalent among World Health Organization (WHO) priority pathogens towards the corresponding antibiotics in India (Mogasale et al., 2021).
Organisms Average Rate of resistance prevalent in India in 2019
E. coli Carbapenem-resistant 45%, 3rd generation cephalosporin- resistance 54%
Serratia spp. Carbapenem-resistant 90%, 3rd generation cephalosporin-
resistance 20%
Shigella species Fluoroquinolone-resistant 75%
Klebsiella spp Carbapenem-resistant 54%, 3rd generation cephalosporin-
manifests superior antimicrobial effects compared to individual parent drugs (Ashajyothi et al., 2016; Christena et al., 2015; Fayaz et al., 2010; Gurunathan et al., 2014; Li et al., 2005). Due to their low toxicity (dosage dependent both in vitro and in vivo), these nanoparticles are preferred in association with broad-spectrum antibiotics. Their high surface area also adds to the overall antibacterial effect (Kulshrestha et al., 2017; Lewinski et al., 2008; Sintubin et al., 2012). It has also been noted that the combined dosage of the drug and metallic nanoparticles can lead to a decrease in the overall administration of medication and toxicity in patients (Kulshrestha et al., 2016). Several active researchers are toiling day and night to find out the exact mode of action of these nanoparticles, but no mechanism has been confirmed yet. In 2010, Banoee et al. (2010) came up with the mode of action of zinc oxide nanoparticles on efflux pumps. NorA pumps in Staphylococcus aureus were taken up for study against the nanoparticles. The zone of inhibition was found to increase for the ciprofloxacin antibiotic when used in combination with zinc oxide. The zone for ciprofloxacin increased by 22% for Escherichia coli and 27% for Staphylococcus aureus (Banoee et al., 2010).
Later Padwal et al. (2014), investigated the synergistic effect of iron
oxide nanoparticles functionalized by polyacrylic acid in the presence of rifampicin antibiotic (PAA-MNP). The target bacterium under study was Mycobacterium smegmatis. When the combinational drug PAA-MNP has experimented, the zone of inhibition got increased by four times when compared to the zone produced by the antibiotic alone. The mode inferred by the scientist was that there was an increase in the intracel- lular concentration of the drug for the new combinational drug. A
ubiquitous substrate for efflux pump (Ethidium bromide) was used to
Salmonella species Staphylococcus aureus
Streptococcus pneumoniae
resistance 63%
Vancomycin-resistant 75%
Methicillin-resistant Staphylococcus aureus (MRSA) 52%, Vancomycin-resistant 1%
Penicillin-resistant 33%
confirm the inferred mechanism in real-time (Padwal et al., 2015). When it comes to efflux pumps, there are two major mechanisms through which they can be hindered. The direct binding of the hindering molecule to the specific site of the pump on the cell membrane. This aids
in blocking the drugs’ flushing out of the cytosol and hence, killing the
Enterococcus faecium Vancomycin-resistant 13%
Enterobacter spp Carbapenem-resistant 57%, 3rd generation cephalosporin-
resistance 7.2%
Proteus spp Carbapenem-resistant 6.7%
Providencia spp Carbapenem-resistant 25%
Others spp. 8.4%–92.9% for Escherichia coli and from 4.1% to 79.4% for
Klebsiella pneumoniae
been a drastic increase in resistance patterns among pathogens in the past five years (Mogasale et al., 2021). Nanoparticles against MDROs have provided ways and mechanisms of mitigating the issue of drug resistance in a very innovative manner (Baptista et al., 2018; HuhandY and Kwon, 2011). Particles in the nano-range have been successfully utilized in microbiology and the pharmaceutical industry for their great therapeutic applications.
Nevertheless, each cellular mechanism of resistance is affected by a different function of the antibiotics. Therefore, it is essential to learn about the common drug-resistant strategies used by the bacteria and how they can be hindered or altered. Among the various techniques available or opted, efflux is a unique system that is made to flush out any unnecessary and toxic substance out of the cell (Sun et al., 2014). These are generally membrane-bound proteins with sites for various substrates to specifically bind on them and activate the target substance’s exclusion process. They also fall under the category of transporter proteins. They are known to have an immense drug pumping out the property (Nikaido and Pag`es, 2012). All the diseases related to the pathogens having an efflux system tend to give rise to strains resistant to multiple drugs. Sometimes they are extensively drug-resistant. However, when these resistant drugs are administered in conjugation with nanoparticles, they have been reported to be very effective.
Many metallic nanoparticles have an inherent antimicrobial activity to hinder these cellular systems of efflux or biofilms. Their combination with conventional drugs has shown synergistic effects with various medications. The combined dosage of antibiotics and nanoparticles
cell. They generally follow a competitive inhibition mechanism to interfere with a given specific efflux pump. Hence, the pump remains dormant and the drug stays inside for longer (Padwal et al., 2014). The second method of hindrance is an efflux system, which would interrupt the efflux kinetics. For example, MexAM-OPrM, a type of efflux pump in
P. aeruginosa leading to multidrug resistance, can effectively be dis- rupted by silver nanoparticles. The efflux pump’s kinetics are disturbed, and the bacterium becomes sensitive to drugs (Nallathamby et al., 2010). They first eliminate the proton gradient, leading to a fall of the membrane potential or reduction in PMF (proton motive force). Finally, the overall driving force required to initiate the efflux activity is terminated (Choi et al., 2008; Dibrov et al., 2002). The major point of concern in this step is that these nanoparticles are so small and reactive that they tend to bind with other membrane components, structures, and pumps. The interaction of nanoparticles with the efflux system exclu- sively is quite complex. In another study, it was reported that copper nanoparticles effectively inhibited norA pumps as they generated copper ions. However, the contribution of copper ions was partially responsible for the overall inhibition of the efflux pump. The major reason for in- hibition was proposed to be the direct binding of copper nanoparticles to the pump. The copper ions aided in the disruption of the efflux kinetics and energy levels, therefore, hindering the activation of the efflux pump (Ashajyothi et al., 2016). When strains of Escherichia coli were grown in different concentrations of copper nanoparticles, they showed a reduc- tion in the membrane potential up to 75 mV (Chatterjee et al., 2014).
The explicit role of nanoparticles in efflux inhibition and the asso-
ciated mechanism is still unexplored. When it comes to translational particles in nano-range for healthcare and medicine, one should be very sure about the synthesis protocol followed, the physiochemical changes caused by the particles both in vitro and in vivo, the kinetics and dy- namics have undertaken along with biodistribution in the body (Boucher et al., 2009). The universal phenomenon of multidrug resis- tance is seen in both gram-positive and negative strains. Each new drug
is potentially exposed to many mechanisms of resistance (Magiorakos et al., 2012). The microorganisms most commonly associated with MDR are Staphylococcus aureus, Pseudomonas aeruginosa, Enterobacter spp, Enterococcus faecium, Acinetobacter baumannii, and Klebsiella pneumoniae. For easy remembrance, they are made into an acronym represented as ESKAPE. The world health organization has declared these organisms a priority for researchers to develop medications and techniques to con- trol their growth and virulence. Among the above-mentioned bacterial genera, three of them are termed as very critical organisms attaining MDR. These are Enterobacter, Acinetobacter, and Pseudomonas (Organi- zation, 2017). Apart from the WHO-recommended genera, researchers have found sufficient MDR species such as Shigella, Escherichia coli, and Proteus mirabilis. The CDC (disease control and prevention organization) has reported that over 2 million people are affected due to ESKAPE every year. The mortality related to the same is also around 23,000 annually (Najafi, 2016). The feature of drug resistance is an outcome of selective pressure that the bacteria undergo. These selective pressures are developed due to illiteracy, lack of awareness, inadequate prescription practices, unauthorized and unsupervised sale of drugs, restricted and unsatisfactory diagnosis, ignorance on the knowledge of drugs and their modes of action, and finally, illegal use of medications (Ayukekbong
et al., 2017).
Each antibiotic has its mode of action on the bacterial cell structure. Various components are targeted to kill the pathogen. They might be the genetic material or the protein production components or the cellular wall and membrane, or the organelles responsible for the overall metabolism of the bacteria. The drugs directly attacking the bacterial cell wall should be designed to bind to specific complementary receptors for initiating cell death. However, those drugs that work inside the cell should be tailored to have the ability to cross the cytoplasmic membrane and reach the target organelle for manifesting its antibacterial effects. An efflux pump is found to create a positive selective pressure for the MDR bacterial strains by letting them thrive in an environment with a reduced concentration of the drug (Masi et al., 2017). These membrane-bound organelles are found in all cells in both prokaryotes and eukaryotes. A source of energy that is mostly chemical in nature is required to activate these pumps and expel the drugs from the intra- cellular portion of the cell. Additional modes can also be associated with the active efflux pump mechanism, which can degrade the active drug through lysis or modification of the drug to bind falsely to other or- ganelles, deviating from the target or tampering with the drug cell permeability to hinder the drug intake into the pathogen. Quorum sensing and biofilm formation also form alternate strategies combined with active efflux to gain slow and passive resistance (Spengler et al.,
2017).
A very expressive and unique exclusion pump will allow the path- ogen to sustain an extended range of compounds. Hence, they evolve into superbugs. Superbugs are known to be the cause of the resurgence of several virulent diseases worldwide. Innovative and smart solutions are on the way to tackle these superbugs. The imperative necessity to find novel approaches in medicine and drug design is pointed out by these superbugs. Newer and fresh modes of action combined with therapies and techniques from all disciplines are the need of the hour (Nikaido, 1994). In this review, various nanoparticles active against MDROs and their possible modes and strategies of antibacterial effects have been summarised. The kinetics and dynamics of combined drugs are also discussed.
2. Bacterial efflux and strategy
Before studying the effects of nanoparticles on the efflux pumps, it is ideal to know the structural and physiological elements of this trans- porter protein system. Alternative measures and treatments are gaining momentum to overcome the scarcity of new drugs to tackle these MDR strains. Use of nanoparticles, incorporation of herbal therapy is trending nowadays. The basic instinct of survival in pathogens has overshadowed
the hope created by the dawn of antibiotics. Few microbes naturally tend to inhibit many first-generation drugs. However, recently, the ability of various previously susceptible microorganisms to gain resistance has caught the eyes of many researchers. The codons encoding for resistance in bacteria are either present in the plasmid, transposons, or the main chromosome. They are reported to be transmitted both horizontally and vertically (Kumar and Varela, 2013). The tendency of the pathogen to adapt effectively to adverse environments and a good capacity of genetic exchange among other strains has made MDR possible. Knowing the exact mechanism of these MDROs will aid in finding a breakthrough to eliminate these pathogens efficiently. Hierarchically, there are two significant types of resistance attained by the bacterial cells. First is natural resistance, also known as intrinsic resistance. This resistance is common to the strains belonging to the same species due to the struc- tural and functional characteristics of the specific strain. The second is clinical resistance, also known as acquired resistance due to genetic mutation in the codons of the target organelles or components of the bacterial cell. The presence of an additional layer exterior to the cell membrane of mycobacteria and gram-negative bacteria results in restricted permeability of any foreign material from outside the cell. Hence, this hydrophobicity attributes to natural resistance (Nikaido,
1994). This structural feature in gram-negative bacteria throws light on
the phenomenon of better resistance in the former than in the gram-positive strains. It is also termed as a passive form of resistance. Even efflux pump activation leads to the same effects. However, when associated with efflux pump, the process of resistance due to membrane permeability is considered as an active process (Poole, 2005). Bacterial strains generally modify the cellular targets to the corresponding anti- biotic to gain resistance. Whatever the antibiotic’s mode of action, each class or generation of antimicrobials has its own set of clinically resistant bacteria. Each resistance arises due to spontaneous mutations at the target site. Later, alterations take place under selective conditions to gain MDR. For example, resistance to rifamycin is possible due to mu- tation in RNA polymerase. The same can be said for quinolone resistance if the mutation occurs in DNA gyrase. Sometimes, conjugation, trans- formation, or transduction also attribute to resistance in many organ- isms. The relocation of the virulent gene from one strain to other is seen in most of the cases. For example, resistance to methicillin is reported to be due to the acquisition of the corresponding gene (mecA) in Staphy- lococcus aureus strains.
Similarly, the van gene encodes for glycopeptide resistance in
Enterococci (Lambert, 2005). These genes can tend to overexpression of the pumps, which leads to hinder various antimicrobial effects of many classes of drugs. Along with the efflux pump inhibitors, membrane permeability and plasmid-based beta-lactamase inhibitors go hand in hand to reinforce resistance in pathogens. Duval and their team inves- tigated Listeria monocytogenes for their mode of resistance. Generally, the protein synthesis components such as ribosomes are blocked. Still, this bacterium tended to split its protein-synthesizing ribosomes to enhance protein synthesis and increase the stability of the cell for survival (Duval et al., 2018). Several reports have shown various unexpected ways of instilling resistance in bacteria.
2.1. Efflux carriers
Active efflux is the prime mechanism used by bacterial cells to remove any unwanted waste from the cell. This also includes antimi- crobial compounds given to restrict the growth of the microbe (Wright, 2011). The efflux system is an energy-based membrane transportation system. It contains sites for specific recognition of complementary molecules. The attachment across the binding site is also very apt.
Once the binding is over, transport across the membrane is initiated. An active receptor or binding site on the pump can be specific to a given drug or may allow multiple binding of similar and competitive compo- nents. When multiple binding and overexpression are feasible for an efflux pump, the characteristic trait of MDR is achieved by microbes (Liu
et al., 2013). If we go into basics, the membrane-bound transporter proteins are categorized into various families. These classifications are based on the cassette used for ATP binding (ABC), cell nodulation for resistance section (RND), extrusion of toxic and multidrug compounds (MATE), resistance to small multidrug (SMR) and superfamily facilitator (MFS). They are denoted as primary structural components of the efflux pump. Some species have additional carriers associated with their pumps. For instance, A. baumannii has a particular carrier for elimi- nating the antibacterial compounds of Proteobacterial origin. They are termed as PACE in short. These carriers are found to be having structural similarities close to the native efflux family SMR (Hassan et al., 2015b). Among the above reported 5 carrier families, ABC uses ATP to remove toxic substances from the pathogenic cell. They are the leading primary carrier in efflux pumps. At the same time, the other 4 carrier proteins use the electrochemical energy existing in the transmembrane. Proton motive force is preferred for RND, MFS, and SMR, while for MATE, so- dium motive force (Nishino and Yamaguchi, 2001) is preferred. Based on the cell membrane composition, the number of pump carriers on the surface varies. Membranes with peptidoglycan layers mostly have sin- gular efflux carriers. They can either be ABC or MFS or SMR. On the contrary, membranes with lipopolysaccharides have severe complex-
ities. Various researchers report a combination of carriers including 600
amino acid alpha helical protein structure is the backbone of MFS car- rier. They are arranged in a helical structure consisting of 12 or 14 moieties. The mode of passage through the carriers is seen to be either symport, uniport, or antiport in nature (Paulsen et al., 1996). The ex- istence of an additional layer in gram-negative strains tends to make the efflux pump adapt to the tripartite structure. Several well-defined carrier pumps of MFS have been documented for MDR gram-positive strains. To name a few, they are QacB, MdfA NorA, QacA, KpnGH, LmrP, CraA, etc. These are present in S. aureus, E. coli, K. pneumoniae, L. lactis, and
A. baumannii, respectively (Schindler and Kaatz, 2016). These proteins have successfully instilled resistance against tetracycline, chloram- phenicol, streptomycin, norfloxacin, cefepime and ceftazidime. The smallest carrier in the lot is SMR. It consists of 100–120 amino acids arranged in membrane-bound helices (4 in number). This smallest car- rier family is subdivided into three, namely, a paired protein, suppressor protein for mutation suppression, and small pumps for multidrug. This carrier is found to be so potent that even a single gene expression has the ability to induce multiple drug resistance in both gram-positive and negative bacteria (Bay et al., 2008; Roca et al., 2009; Srinivasan et al., 2014). In some strains such as E. coli, SMR has 110 amino acids. The SMR protein is here termed as EmrE protein, and it aids in the expulsion of ammonium and acriflavine compounds (Banigan et al., 2015). Some more examples to quote for SMR are EbrAB, QacC, and SepA. They belong to B. subtilis and S. aureus, respectively (Schindler and Kaatz, 2016).
RND proteins are a special class of carrier proteins that work devoid
of the physiochemical trait of the toxic material. They are not altered by the amphiphilic or hydrophilic nature of the target substance. Hence, they are used for the exclusion of a broad range of molecules. They are comprised of 100 and more residues of amino acids. RND proteins associate with the outer, inner, and periplasmic spaces of the membrane due to their tripartite assembly. They are generally multimeric pumps. MexB and AcrB are proteins associated with the cytoplasm on the inner membrane. MexA and AcrA are periplasmic proteins that make up the body of the carrier protein. OprM and TolC are the proteins associated with the outer membrane. Hence, the overall structure of RND is consolidated. Some well-known RND carrier proteins are MexABOprM and AcrAB-TolC. They are found in P. aeruginosa and E. coli, respectively (Nishino and Yamaguchi, 2001). The ArcB range of carrier protein has an affinity to various medicines such as b-lactams, phenicol, fluo- roquinolones, cyclin, and several solvents and detergents (Beketskaia et al., 2014). The unique structural setup of the whole RND prefers low molecular weight molecules (Takatsuka et al., 2010). It has been sug- gested previously through various hypotheses that each sub-class of
RND undergoes periodical changes in its conformation. Complimentary alterations further influence these changes to the nearby units. Simi- larly, deactivation of any one of the subunits will lead to total loss of the entire RND protein function. This typically happens if there is a delay or problem in proton transmission in the subunits (Takatsuka and Nikaido, 2009).
Lastly, Cassette for ATP binding (ABC) is an energy-dependent pri- mary carrier protein harbored in the trans-membrane region of the cell. It is found in all types of living cells (Davidson et al., 2008) and has an affinity to many target molecules. Its substrates can be sugars, peptides, metal ions, etc., and it is the largest protein carrier in the family of efflux pumps. The most significant ABC protein has around 1200 residues of amino acids. These large moieties are arranged systematically in 4 do- mains. Out of 4, two domains are hydrophobic. They aid in recognition of the target and then the transport out of the cell. These two domains are found deep in the trans-membrane space. The other two domains are exposed in the cytoplasmic region. They bind with ATP and break it down by hydrolyzing it (Schmitt and Tamp´e, 2002). In a strain with lipopolysaccharide layers, the primary carrier protein (ABC) is associ- ated with the adapter protein in the periplasmic space and the protein on the cell’s outer membrane. This interaction aids in letting the foreign molecules pass through the membrane and get expelled out into the extracellular portion of the cellular lining. An ideal example to quote is the well-known MacAB-TolC protein of E. coli. It is unidirectional in operation and ATP-driven (Gupta et al., 2011). MATE carrier protein causes hydrophilic medications inactive in most pathogens. They harbor approximately 450 residues of amino acids. They also have 12 struc- turally similar membrane segments. The most common medications that are made inactive due to this carrier efflux protein are aminoglycosides and fluoroquinolones (Putman et al., 2000). Most organisms have MDR because of the presence of MATE carrier protein that generally uses sodium motive force. However, some unique bacteria such as
A. baumannii, P. aeruginosa, and S. aureus use proton motive force. The genes associated with these organisms would be AbeM, PmpM and MepA, respectively (Kaatz et al., 2005; Kuroda and Tsuchiya, 2009).
2.2. Efflux and quorum sensing
Efflux systems work hand in hand with other forms of mechanisms that cause resistance in bacterial species. They have a very vital role in signaling between cells and between molecules and cells. This leads to the formation of biofilms in pathogens. To effectively tackle therapeutic resistance in microbes, it is necessary to inhibit both efflux and quorum sensing systems of a virulent pathogen. This will result in restriction of biofilm formation. Several researchers have proposed the synergistic effect of efflux expulsion systems on quorum sensing (Bay et al., 2008; Hassan et al., 2015b; Nishino and Yamaguchi, 2001; Roca et al., 2009; Srinivasan et al., 2014). When resistance increases in a particular strain, it will by default increase the population of the concerned species in the given environment. As the efflux system keeps flushing contents into the surrounding environment, specific inducers are introduced into the extracellular region of the cellular population by the virulent strains. As bacterial cell communication is high-speed and simple, the other cells in that area adapt to the virulent pathogens’ resistance. Hence, resistance is spread to the given population of bacteria. These autoinducers can accumulate in the surroundings and enhance population density, thereby leading to alterations in the genetic makeup of the organism. The genes associated with quorum sensing always trigger expression towards the population’s phenotype surviving in proximity. Many
functions are controlled and monitored by quorum sensing. These
include antibiotic secretions, bioluminescence, production of spores, biofilms, etc. Even virulence initiating in bacteria is controlled by this phenomenon, as it manages the respective factors and proteins that cause resistance to drugs (Novick, 2008). Each strain of bacterium has its own mechanism of quorum sensing followed by unique components for regulation of its physiological functions. There are three basic guidelines
that every quorum sensing system follows. Initially, inducers are generated automatically from the bacterial cells. These will start cellular communication while signaling the neighboring species. When the cellular density in an area is low, the inducers will diffuse away from the parent cell and are present in low concentrations to be detected by any technique. As the population of the bacterial species increases, the combined and elevated production of inducers from each cell leads to a higher concentration of the same in a given localized area. This con- centration is high enough to be assayed, and a suitable response is documented based on the scenario of the cells (Kaplan and Greenberg, 1985). The second step in the process is the reception of the inducers by suitable and specific receptors. These are located facing the cytoplasm or are anchored on the cell membrane. Finally, the genes associated with the cooperative environment between all the strains are activated by inducers (Kaplan and Greenberg, 1985). The only legit reasoning for the relation between efflux and quorum sensing is that the efflux system can aid in flushing the inducers and the toxic and undesirable wastes into the outside environment.
Nevertheless, a detailed relationship is still unknown and undocu-
mented (Waters and Bassler, 2005). The inhibition of the efflux pump will hinder the concentration in the external environment and, hence, adversely affect quorum sensing (Xu, 2016). Suppose blockers for efflux systems are utilized effectively. In that case, the whole series of reactions and pathways are altered and will reduce undesirable film formation and reduce the transfer of resistance patterns between species. Even aggregation of neighboring cells is inhibited by the efflux pump’s detrimental conditions (Xu, 2016). Metal-based nanoparticles have shown the tendency to restrict and hinder efflux systems in synergy with conventional antimicrobials. The ATP pathway for energy production is predominantly disrupted (Shah et al., 2006).
3. Alternate strategies for MDR treatment
Resistance in drugs has been reported for almost all the medications used for veterinary and human welfare. Therefore, it is essential to develop new ways and techniques to conserve the given resources of conventional medicine and make them as efficient as possible to face the current MDR crisis (Gonza´lez-Bello, 2017; Wright, 2016). Most of the drugs used are generally seen to be plant-based derivatives or chemical derivatives of the same. As of now, there are two exceptional drugs that can work on any new strain of bacterium. One is ACHN-975, and the other is Brilacidin. The former is a first-line emergency medication as an initial defense against most infectious species. The latter is still in clin- ical trials. The mode of action is seen to be LpxC inhibition. This enzyme is responsible for lipid A synthesis in pathogens. The current microbial and pharmaceutical research trend is to come up with the measures and components that can address the virulence/resistance issue of the microorganism and eliminate the bacteria as a whole. When a therapy or technique does not manipulate the growth and death of a microorganism but alters only the virulence, it is considered as an antipathogenic drug and will nullify the infectious nature of the pathogen. Therefore, the host doesn’t get affected by the disease. The altering genes in the pathogenic strains can also generate antipathogenic effects. The severity of the disease and symptoms can be tailored easily to provide relief to the patients (Israil and Chifiriuc, 2009). Most of the efflux blockers also work towards the antipathogenicity of the organism. These compounds are much better sought for in comparison to designing new-generation drugs. Sometimes, they work towards breaking down the communica- tion signals between the population of microbes. This induces anti- pathogenicity without being lethal to any cell. The outcome of this breach in communication between the cells would cause a reduction in selective pressure. This would lead to hindrance in the resistance of the pathogen (Waters and Bassler, 2005). It also manifests that the use of other techniques like gene manipulation also has a positive effect in controlling the virulence of a microorganism. Other techniques that might help are immune-based therapy and vaccine-based therapy. These
techniques can also keep in check the feasible mutations that occur in the genetic makeup of an infectious agent. Therefore, controlling the resistance pattern in the pathogen (Lazar et al., 2005).
To have targeted delivery by nanomaterials, it is essential to know about the new site for the attack on an organism. This will aid in designing new medicines and innovative nanoparticles to target com- ponents that have enhanced antipathogenic and bactericidal effects. Before fabrication of nanoparticles for targeted delivery, it is essential to have a cumulative and detailed study of the pathogen and its environ- ment using molecular biology, bioinformatics, proteomics, etc. This will generate a functional and knowledgeable database relating to the in- fectious agent and its modes of action, which in turn can pave the way for the required nanomaterial for treatment (Piddock et al., 2010). To gain information on the chemical aspects of the proteins and pathways associated with the virulence of the microorganism, chem-informatics can be opted. It provides a wide variety of data on ligand binding and its kinetics and relationship studies. It also throws light on the structural changes and conformational effects based on activity. These studies will provide a good screening of potential compounds similar to commercial drugs based on reasoning, logic, and experimental evidences (Hammami and Fliss, 2010). Similar research was performed in gram-negative strains by a group of scientists. They found an innovative aminoglyco- side that was designed to target and inactivate its corresponding enzyme
in the cell (Sardari and Dezfulian, 2007). Some minute and low molec-
ular weight peptides with antimicrobial activity are also devised in labs for the same purpose. They can be extracted and tailored naturally or made synthetically too. Generally, their weight is kept to a maximum of 50 kDa. They are altered and created to attack cellular membranes and change the genetic makeup of the pathogen. They also interfere with the infectious agents’ metabolic pathways, posing a suitable alternative treatment method for various infectious diseases (Ramirez et al., 2013). Nonetheless, the fabrication of these novel proteins is an expensive affair, significantly when escalated to scale up to commercialization processes. Their sensitivity to the host pathogen’s proteolytic enzymes can lead to the peptide breakdown before reaching its target site. Their overall toxicity to the human body also needs to be considered before administering to patients. These peptides show enhancement in their features and properties when used in combination with nanotechnology. Then, nanosized or encapsulated forms can avoid degradation due to the host enzymes. Their availability and absorption are increased. The overall toxicity is also reduced significantly (Carmen Chifiriuc et al., 2014; Peters et al., 2010). However, these techniques need high in- vestments and funding. They are also in need of several sophisticated equipments for fabrication and characterization. Hence, their applica- tions are limited, and commercialization in the market becomes an issue.
4. Natural efflux pump inhibitors
Most of the efflux system blockers are in the nano-size range. Poly- phenols of natural origin have always been a great source of efflux blocking compounds. They are stable in structure and chemically, very functional, and unique. It also harbors several metabolites of secondary origin with numerous pharmaceutical applications. Previously, many reports have been published on the use of herbal extracts in all organ- isms. Both gram-positive and negative species have successfully experimented. They also can restore the lost susceptibility to commer- cial antibiotics. The concentration of the drug inflow into the host is also elevated thereby, having an enhanced bactericidal effect. Few families are very much well known for their remarkable bioactive compounds as potential efflux system blockers of all the plant families available. A few potential plant families are Zingiberaceae, Convolvulaceae, Apocynaceae, Lamiaceae, Berberidaceae, Fabaceae, and Cucurbitaceae. Since being generally consumed as foods by the general crowd, their incorporation in medicines as efflux blockers is of growing interest. Many fruits, seeds, vegetables, and spices are investigated every day all around the world. A few active ingredients introduced in nanomaterials to enhance the efflux
systems are piperine, farnesol, ellagic acid, resveratrol, thymol, gera- niol, p-coumaric acid, theobromine, etc.; their derivatives and metabo- lite forms are preferred in combination with nanomaterials. Among these are essential oils that are aromatic in nature. They possess a chemical gradient that has an affinity towards efflux blocking. Geraniol, carvacrol, farnesol, and thymol are well-known aromatic herbal plants with active components for prospective nanomaterial conjugation and utilization. They can inhibit the efflux system in 4 major modes. They either tackle the proton motive forces or the sodium motive forces. Sometimes, they act as competitive compounds for the binding sites on the efflux pumps, hence, keeping the pumps in an inactive state. Few molecules follow inhibition in a non-competitive way. Some other modes opted apart from these steps are disruption of the gradient across the membrane of the host. The factors associated with pathway regu- lation can also be hindered if the genes responsible for the same are blocked. Hence, the efflux expression decreases. The energy source ATP can be broken down or interfered by functionalized nanomaterials, which might increase the permeability of the liposaccharide layer. The drug crossing across the membrane increases, and the antibiotic effect is also enhanced. Direct change in the conformation of the pump is also feasible to get the required results to handle virulence in strains (Piddock et al., 2010).
5. Other sources
Apart from plant sources, several microorganisms can also produce compounds and by-products with similar activity towards the efflux pump (Stavri et al., 2007). Streptomyces vellosus produces specialized compounds such as EA-371 d, EA-371a, etc., that have a restriction ef- fect on the efflux system of P. aeruginosa (Lee et al., 2001). Some strains have mutated down the years to produce novel metabolites to overcome the resistance attained by other strains. Termites extracted with alcohol produce metabolites that have antibiotic properties. They reduce the minimum inhibitory concentration of organisms like E. coli and Staph- ylococcus aureus (Coutinho et al., 2009). Neonectria discophora is refined and extracted to produce antimicrobial compounds to treat infections caused due to Staphylococcus aureus and T. rubrum. The active in- gredients produced are derivatives of ilicicolinic acid (Chaves et al., 2015; Sorres et al., 2018). The process of extraction seems to be tedious for organism sources when compared to plant sources.
5.1. Metallic sources
Many metal-based nanoparticles play a crucial role in efflux inhibi- tion. The most common metal-based nanoparticles are discussed in detail in the below sections.
Chitosan - This is a polysaccharide with a linear structure and linkages. It has a beta (1 ? 4) linkage between its monomers. One deacetylated unit of glucosamine binds with an acetylated form of glucosamine. Chitosan is extracted from a natural polymer called chitin (Kumar, 2000). The special feature of this polymer is its compatibility with biological tissues and environment-friendly nature. It is potentially used as a drug carrier agent. Targeted and sustained release is feasible by this polymer. Drug temporin B has been successfully encapsulated in the nano-carriers of chitosan to enhance its antimicrobial activity (Piras et al., 2015). This polysaccharide in acidic environment attains a posi- tive charge that could be of great harm to the oppositely charged genetic material. Even the cell membrane is negatively charged; hence, it has a great affinity to this polymer. When a detailed study was performed on gram negative strains for the effect of chitosan nanoparticles on them, it was found that these nanoparticles instilled a heightened affinity to the inhibitor. These are generally found in the growth media in the form of special dyes and micronutrients. There is an overall loss of the barrier function due to the affinity binding by the chitosan nanoparticles on the surface of the cell membrane. They cause extensive surface abrasions and cover the periphery as a sheath to block the vesicular functions
(Helander et al., 2001). They also have shown evidence of binding with the cellular genetic material to hinder the essential transcription and translational processes (Pelgrift and Friedman, 2013). In 2009, the minimum inhibitory concentration of sulfamethoxazole was effectively reduced to a great extent when used along with chitosan in the nano range. The decrease was seen to be five folds. Pseudomonas aeruginosa has an over-expressive efflux system (MexEF-OprN). This carrier is affected the most by these chitosan nanoparticles (Tin et al., 2009). The suggested reasoning provided by the researchers is that the combina- tional synergy between the drug and chitosan nanoparticles attained a change in the expression of the efflux carriers.
Silver – this metallic compound has been in practice to treat various illnesses since ancient times. It is very much well known for its antimi- crobial activity. Greeks in their ancient history had reported the use of nanocrystals to treat a broad spectrum of pathogenic species. Approxi- mately 150 different strains of bacteria are effectively treated using silver nanoparticles (Mijnendonckx et al., 2013). Derma treatment for burns, soothing drops for eyes, and tending to chronic wounds have used silver for many centuries. Even trachoma has been avoided with great precision due to the utilization of this metal (Lemire et al., 2013). Catheters used in body bags have been lined with polymers that are conjugated with silver. The antimicrobial activity of the metal leads to inhibition of biofilm formation on the walls of the catheter. Even topical formulations for skin ailments contain silver to a greater extent. Most of the formulations of silver are generally in the nano range (Maillard and Hartemann, 2013; Prabhu and Poulose, 2012a). Although the microbial inhibition activity of silver is well experimented with and documented, the mechanism opted by the same is still unknown partially. Many re- searchers have inferred that the mode of action for silver is actually a combination of several mechanisms. One of the most common modes is the passage of metallic nanoparticles across the membrane. This will lead to the accumulation of the same in the intracellular compartments.
The activation of the reactive oxygen species is triggered to produce free
radicals. This leads to the shutting down of the essential enzymes, finally breaking down the genetic makeup of the host-pathogen. This also hampers the activation and working of efflux pumps (Prabhu and Pou- lose, 2012a). The ionic form of silver has a great affinity towards most moieties present in a cellular membrane. The thiol residues of the NADH bind with the silver ions along with the oxidoreductase of quinone. While binding, the structural integrity of the wall is brought down (Xu et al., 2004). This results in the block in the energy pathways of the pathogen, hence, adversely affecting the host bacteria. They nullify the DNA and RNA building blocks by the ROS mechanism. This hinders the central dogma of the cell. Even resulting protein and lipid conformations are damaged (Mijnendonckx et al., 2013). Two research teams have reported their novel synthesis of single silver metal nanoparticles. They were spherical and had surface plasmon resonance that was localized to a single spectrum of silver nanoparticles. Their experimental hosts were Pseudomonas aeruginosa and Bacillus subtilis. The effect of size on the kinetics of the ABC carrier of efflux pump was studied for both patho- gens. The study was kept real time and used two very common in- struments for characterization: a spectrophotometer and an optical microscope in dark field mode. The resolution was kept in nano range. They observed and inferred that the smaller the size of the nanoparticle
the better the retention of the particle in the host-pathogen. The larger
particles got easily flushed out with time. Therefore, they were able to give evidence to the fact that the size of the nanoparticle would influ- ence the efflux pump kinetics, thus, affecting the resistance property of the bacteria (Lee et al., 2010). Similarly, size effect of nanoparticles and attainment of MDR in Pseudomonas aeruginosa were studied successfully in 2010. The transporter exclusively under study was the MexAB-OprM. When observed in nano-range resolution, the wild strains manifested higher retention of nanoparticles in the cells. The cells having the multidrug transporter in an overexpressed state showed a lower reten- tion of the nanoparticles. When the transporter was blocked using an inhibitor, the results became favourable and reversed than previously
reported. Most commonly reported and used efflux blocker is CCCP (Carbonyl Cyanide m-Chlorophenylhydrazine), was also used as control in the experimental studies (Nallathamby et al., 2010).
The above strain of the bacterium was also studied by another group of scientists with aztreonam and chloramphenicol antibiotics. Three types of strains were studied for the same transporter. One being wild type, other being a mutant and overexpressing, known as nalB-1 and the last being a mutant that didn’t express the gene MexAB- OprM. The latter was depicted as ?ABM. The controlling parameters and kinetics of the efflux carrier MexA-MexB- OprM were studied for both the mutants using both the drugs. In the absence of the aztreonam drug, the accu- mulation of nanoparticles was seen to be at a lower frequency inside the bacterium. As the time-based data were collected for the same, the number of retained nanoparticles didn’t differ over the course of the experiment for nalB1. Instead, in ?ABM, the silver nanoparticle accu- mulation increased with time. When the aztreonam drug was adminis- tered, both the strains showed enhanced retention of nanoparticles over the course of the study. The increase was found to be very much pro- portional to time. When the study was closely monitored and inferred, it manifested that the carrier MexA-MexB-OprM played a vital role in retaining the nanomaterial in the cell. The synergy of the drug and the silver nanoparticles was very well depicted. The drug aided in bringing down the membrane integrity and the silver nanoparticles caused the
toxicity as well as affected the efflux system of the host (Wijnhoven
et al., 2009). Thus, the efflux system was summed under the pressure of multiple substrates (both silver nanoparticles and drug together). Similar results were seen for chloramphenicol also. The level and degree of accumulation in the intracellular space influenced the expression and kinetics of MexAB-OprM. The ?ABM mutant strain accumulated the largest number of silver nanoparticles in comparison to the nalB-1 strain. In the absence of the chloramphenicol drug, the accumulation and retention of nanoparticles were found to be good in both wild type and ?ABM. However, the nalB-1 had very low accumulations as their MexAB-OprM transporter was still functional and expressive. The rate of accumulation elevated up to 10 folds for a mere concentration of 25 µg/mL of the drug (chloramphenicol) in the wild and ?ABM mutant species. Nonetheless, in nalB, it didn’t change at all. The nanoparticles accumulate on the cell membrane of the bacterial strain and does per- manent damage to the structural conformation of the wall. This led to improper working of the efflux system (Xu et al., 2004). Hence, the resistance attained by any pathogenic species can be altered by silver nanoparticles in combination with the parent drug (Kyriacou et al., 2004; Li et al., 2005).
The synergistic effect of ß-lactam drugs and silver nanoparticles were studied by Li et al. (2005)). They found that the combination of silver nanoparticles with drugs was found to be more lethal when compared to their individual effects. They hinder the respiratory pathway of the in- fectious host and destroy the permeability of the membrane (Shahverdi et al., 2007). This was found to be a parallel study to the above- mentioned studies. They worked with stronger medicines such as van- comycin, penicillin G, clindamycin, amoxicillin, erythromycin, etc. When used in conjugation with silver nanoparticles, their dosage of administration also decreased. The toxicity is easily monitored and controlled for the conjugated form of the drug (Mohammed et al., 2011). Proper evidences for cellular membrane and proton motive force dam- age have been reported via transmission electron microscopy and pro- teomic analysis (Li et al., 2011; Lok et al., 2007). In china, a group of scientists worked on the possible mechanisms of silver nanoparticles inside the cell. They inferred that the over accumulation of the nano- particles led to condensation pressure on the chromosomes. The con- tents lost their ability to replicate and move freely into the surrounding environment. The host studied in this experiment was Staphylococcus aureus. The dehydrogenase enzyme that takes part in the respiratory and energy generation process is altered to have a reduction in its functional activity (Schairer et al., 2012).
Nitric oxide – recently the use of nitric oxide nanoparticles for
bacterial growth and sustenance is of great interest to scientists. They seem to follow a wide range of modes to act against pathogenic strains. The primary mode would be to form reactive species of nitrogen oxide moieties that would interact and bind with many cellular proteins and cause disruption to the organelles. These reactive nitrogen oxide species have the tendency to attach with the DNA and RNA materials to initiate damages to the repair enzymes of the genetic materials. The respiration process of the pathogen is paused and affected as the reactive nitrogen oxide attaches to the zinc metalloproteinase enzyme (HuhandY and Kwon, 2011). This results in reduction in the efflux activity. Even small quantities of nitrogen oxide nanoparticles are found to be effective against some of the major MDR strains such as Klebsiella pneumoniae, methicillin resistant Staphylococcus aureus, Enterobacter faecalis, Escher- ichia coli, Pseudomonas aeruginosa, etc. The spectrum of action of nitro- gen oxide species is very broad covering most of the pathogenic strains, be it gram positive or negative species. The membrane bound lipids and proteins are bound to alter the integrity of the plasma barrier, which in turn affects the efflux systems. However, the amount of virulence is noticed more in gram negative strains when compared to the gram positive strains (Raafat et al., 2008). Similar mechanism is noticed for nitric oxide nanoparticles on both gram positive and negative strains.
They adhere to the components of the cell membrane and alter the
integrity of the barrier. Hence, they are easily transported into the host. Zinc – The mode of action of zinc nanoparticles is found to be almost similar to that of silver. They breakdown the membrane integrity and generate reactive oxygen species. These species will neutralize the ge- netic material of the bacteria and further tend to alter the conformations of lipids, proteins, etc. (Ghasemi and Jalal, 2016). Till date, zinc based nanoparticles are used in combinations with various commercial med- icines to enhance their efficacy and overcome resistant strains at ease. Amoxicillin, erythromycin, cephalexin, cefotaxime, vancomycin, tetra- cycline, cloxacillin, gentamicin, clindamycin, ceftazidime, ampicillin, erythromycin, oxacillin, streptomycin, etc. show a great synergy when used in combination with zinc oxide nanoparticles (Venubabu Thati et al., 2010). Some have reported that based on the drug features and composition, the effect of nanoparticles varies based on the overall antimicrobial activity of the particle. Drugs such as penicillin G and amoxicillin manifested reduction in antibacterial activity against Staphylococcus aureus. However, ciprofloxacin, on contrary, had an in- crease in its antibacterial activity when used in combination with zinc oxide nanoparticles. The efflux system (NorA) falls short of energy as the whole pathway is disrupted by the nanoparticles. The highly resistant fluoroquinolones are made susceptible again via these nanoparticles (Couto et al., 2008). The membrane characteristic protein Omf also has a
great affinity towards zinc oxide nanoparticles. They aid in persistent
passage of quinolones through the membrane barrier. This leads to an enhanced absorption of the drug inside the cell (Banoee et al., 2010). Efflux setup is also hindered simultaneously. The formation of com- plexes between the drug and the zinc oxide nanoparticles also favours the abovementioned mechanisms. These were studied and documented in A. baumannii (Ghasemi and Jalal, 2016).
Gold- Aurum has its application in medicine since civilization. It has a very few effects on bacteria. When combined with potent drugs, it does wonders. Some medications used in combination with gold nano- particles are mecapto ethylamine, 4, 6-Diamino-2 Pyrimidine thiol, 3- mercapto, p-Mercaptobenzoic acid, propyl sulfonate, etc. They tend to attach and form complexes with the transfer RNA and block the trans- lational process of the subunits responsible for the efflux pump. Due to decrease in the structural units of the efflux pump, the whole system will collapse and resistance will be controlled. The ATP associated enzyme activity is also altered; hence, the energy supply goes down for the efflux pumps. Enterococcus species resistant to vancomycin, E. coli resistant to vancomycin and Pseudomonas species resistant to methicillin, etc. are treated with great care by gold nanoparticles (Hayden et al., 2012). When compared to other inert metal-based nanoparticles, gold is found to be very much superior in its design and applications. They are found
to be very much easy to be monitored when it comes to shape and size of the nanoparticle. Gold nanoparticles are functionalized easily using different ligands for different functions. They are stable and are biocompatible to living beings (Rosi et al., 2006). Gold is a very convenient platform used by numerous researchers to explore its po- tential in medicine. Its conjugation experimented with nucleotides, proteins, carbohydrates, single molecules, etc. is of great interest for scientists. These miniature moieties have potential biological applica- tions and also, aid in designing fundamentals for the fabrication of specialized materials for diverse fields (Shahverdi et al., 2007). Particles that have multiple valences in the nano range can be used for surface modifications of gold. They are an ideal research model for testing polymer-based nanomaterials too. These remarkable gold nanoparticles are 100 times tinier than a general bacterium. When observed in solu- tion, they exhibit a totally contrasting dimension when compared to other counterparts. Gold nanoparticles in conjugation with vancomycin have found to be elevating the bactericidal activity of the drug against its resistant strains such as Enterococcus. Their mode of action is depicted to be polyvalent inhibition of the efflux (Gu et al., 2003). N-acetyl lac- tosamine along with nanoparticles of gold has proven to be a good combo to restrict the attachment of enteropathogenic E. coli to the cell
linings. Hence, their localizations are also hampered (Hyland et al.,
2006). Corynebacterium is treated well with gold nanoparticles that create perforations in the cell wall of the pathogen. Later, they accu- mulate in the intracellular space and cause further damage to the efflux pump too (Mohamed et al., 2017). Gold nanoparticles functionalized by pyrimidine have a peculiar property of sequestrating the mineral ions such as magnesium and calcium present in the cell. This process results in damaging the bacterial wall and enhancement in permeation of the same. Even, the nucleic acids get transported out of the cell. Internali- zation of the nanoparticles into the cell leads to malfunctioning of the protein synthesis machinery that might affect the efflux pump system (Zhao et al., 2010). Therefore, the susceptibility to vancomycin in- creases in Enterococci when gold nanoparticles are used along with it. When the same conjugation is experimented with ampicillin drug it showed antimicrobial activity towards a broad spectrum of species. When ampicillin was used alone against most of the gram positive and negative strains, they were found to be resistant to the medication (Brown et al., 2012). The malfunctioning of the efflux system due to blocking by the gold nanoparticles and also, multivalent orientation of the drug to the pathogen are possible inferences that the researchers were able to point out. They were also seen to alter the tRNA of the translational pathway so that it could not bind to its required subunit and hence, collapsing the whole protein setup. Similar study was
attempted by Khambesh and team and they received the same results for
gold nanoparticles as reported above (Khameneh et al., 2016; Zhao et al., 2010).
Magnesium– the nanoparticle form of magnesium is generally its oxide form. Magnesium oxide nanoparticles generate reactive species of oxygen that tend to degrade the infectious host cell. However, few have reported that the magnesium oxide nanoparticles can have a reverse effect on the efflux system of the virulent strain (Pelgrift and Friedman, 2013). When the halogen group molecules are used in combination with magnesium oxide nanoparticles, the efficacy of the overall composite is enhanced to a great extent. Molecules of fluoride, chloride, bromide etc have a good affinity of adsorption on these oxide nanoparticles. Once reacted, they form their respective magnesium fluoride, magnesium chloride and magnesium bromide nanoparticles. Studies have reported that these compounds do have bactericidal effects against many path- ogenic species such as Staphylococcus aureus or Bacillus subtilis (Hajipour et al., 2013).
Copper – Nanoparticles of copper are mostly preferred for elec-
tronics or material science purposes. Their utilization in efflux pump inhibitors and microbial control are quite scarce. Several researchers are now exploiting these nanoparticles for potential applications in MDR strain control. Many mechanisms and modes are proposed by various
studies. Sometimes, they tend to directly bind to the bacterial membrane and cause damage. They also have the ability to produce free radicals that have an adverse effect on the cellular organelles of the host path- ogen. Crosslinking of the copper nanoparticles with genetic materials such as RNA and DNA results in breakage and disorder in the whole structural setup of the chromosomes. Articles are also published on the conformational changes in the helical orientation of the DNA due to mutations by the nanoparticles. These mutations are seen to be either deletions or insertions that hinder the optimal expression of the codons. At times, these displace the vital metals associated with the cellular proteins and subunits (Christena et al., 2015). Few strains show a higher degree of susceptibility when compared to others. For example, Pseu- domonas aeruginosa is not influenced much by copper nanoparticles but strains of Staphylococcus aureus, Enterococcus faecalis and Escherichia coli are greatly affected by the presence of copper nanoparticles (Bagchi et al., 2013). Several strains are concentration dependent for the bactericidal activity of the copper oxide nanoparticles. The minimum inhibitory concentration for each species varies with the different con- centrations of nanoparticles administered. The efflux system of Staphy- lococcus aureus is found to be inhibited if a 0.032 mM of copper nanoparticles is introduced into the culture. However, bacterial patho- gens such as Pseudomonas aeruginosa needs nearly double the concen-
tration of the nanoparticles to hinder the efflux pump’s functioning.
When compared to conventional antibiotics, copper has better features as an antibacterial agent. The minimum inhibitory concentration of copper nanoparticles is reported to be far better than ciprofloxacin against methicillin resistant Staphylococcus aureus (Christena et al., 2015). The carrier associated with the resistance pattern in Staphylo- coccus aureus is the Nor A efflux carrier protein. These nanoparticles block them and make their functions inactive (Couto et al., 2008). In the same study, it was observed that at lower concentrations of the nano- particles, copper aided the bacterial population to stop the production of biofilms and enhanced the sensitivity pattern in them (Christena et al., 2015). When lower dosages of copper nanoparticles are provided to the bacterial cultures, the regulators responsible for response to multiple antibiotics (Mar R) get changed. The structural moieties of MAR R have residues of cysteine in them. When copper comes in contact with them, they form tetramers by means of disulphide bonds. These tetramers stop the whole process of protein synthesis associated with biofilm forma- tion. They also have shown a peculiar trait as a signalling agent in Staphylococcus aureus. This signalling alters the expression of the genes that are responsible for the external polysaccharide layers. The efflux pump blockers will also have a dual function of inhibiting biofilm for- mation too. Even films produced due to MFS and RND carriers can also
be abolished with a help of a simple efflux blocker (Hao et al., 2014).
The mediation in copper-based therapy is actually done by copper (II) ions. Only a part of its pharmaceutical activity is attributed by the nanoparticle form of copper.
Iron – Ferrous and ferric nanoparticles have several potential ap- plications in the field of medical microbiology. They have the ability to retain and radiate heat. Hence, they are preferred for hyperthermia treatment on bacteria. They also are very suitable candidates for tar- geted drug delivery and therapy (Chertok et al., 2008). When it comes to imaging, it is a well-known image contrasting agent for MRI (magnetic resonance imaging) (Xing et al., 2013). The basic antibacterial action of iron nanoparticles is because of the oxidation of the intracellular com- ponents of the parent cell. The hydrogen peroxide generated in the pathways tends to give away hydroxide and ferrous ions. They are the stepping stones to the final reactive oxygen species that cause stress in the cells and disrupt the cellular components (Tran et al., 2010). Based on the presence of atmospheric oxygen, the effects of iron nanoparticles and their oxidative effects vary. Under anaerobic circumstances, the antibacterial activity is much more lethal and harsher than in aerobic or atmosphere saturated environments (Lee et al., 2008; Sies, 1997). The administration of functionalized iron nanoparticles with tuberculosis medications like rifampicin has aided in the treatment of TB resistant
strains of M. smegmatis (Padwal et al., 2014). They cause damage to the protein subunits and chromosomes of the bacterium. This might lead to malfunctioning of the efflux pumps. The only saving grace of theses nanoparticles when compared to other nanoparticles is the ease of fabrication and are inexpensive.
Calcium – this is a mineral that has its major applications in the field of implants and prosthesis. They cover dental and orthotic based aids and aesthetics. The calcium composite used in dentistry is made up of fillers in the form of glass for reinforcement in a matrix composed of polymer resin and finally, a coupling agent from silane. They have good mechanical and aesthetic properties, used in dental restoration and surgeries (Lim et al., 2002). When utilized for long, they have reported the formation of biofilms and plaque growth (Drummond, 2008). To increase the longevity of the implants, it is essential to load the matrix with bioactive agents to combat the microbial growth and resistance. Nanoparticles of calcium loaded with biologically active agents are used in dental implants to avoid recurrent infections, cavities and good me- chanical load strength (Yoshida et al., 1999). Calcium nanoparticles can be paired with various antibacterial agents to reap several benefits. To name a few, the antibacterial agents associated with calcium nano- particles are silver, quaternary ammonium salts, phosphates, etc. (Tanagawa et al., 1999). The phosphate forms of calcium release the ionic form of calcium along with phosphates in supersaturated con- centrations. These ionic varieties help in repairing the cavities in the tooth and fill up the lesions. The combination of silver, quaternary ammonium salt in nano range and phosphates of calcium has shown
restricted metabolic performance of the bacteria. The combo hinders
colony accumulation near the teeth and film formation of S. mutans. The performance of the trio seems to be more promising when compared to two of the most commonly used commercial counterparts (Cheng et al., 2012). Some composites of calcium are pH dependent for their anti- bacterial activity. The hydroxide of calcium when used as dressing for intracranial lesions, manifested a potent bactericidal effect at alkaline pH around 12–13 (Bystro¨m et al., 1985). The presence of special active agents can also influence the performance of the calcium composites towards the bacteria. A group of scientists worked on the effect of calcium-based nanocomposite on Enterococcus faecalis. The effect of calcium hydroxide along with nanoparticles of chitosan with very minute amounts of functional disinfectant was seen on the efflux pumps of the same. There was no subsequent depletion in the film formation and even after 24 h, there was no breakdown on the film layers. The results didn’t differ even when different concentrations of calcium hy- droxide were administered. When disinfectant was employed in increased concentrations, they easily inactivated the 48 h old biofilm of Enterococcus faecalis. Introduction of additional inhibitor in the form of calcium hydroxide composite at minute concentrations, showed enhancement in the antimicrobial efficacy. At higher concentrations, this ability was lost (Upadya et al., 2011). However, many researchers don’t find this calcium nanocomposite to be effective against Entero- coccus faecalis for tooth related queries (Distel et al., 2002). The property of killing or hindering the growth of microbes is due to the calcium ions. In gram positive strains, their barrier membrane consists of carboxylate and phosphate moieties, which form binding sites for calcium ions (Hancock, 1997). There is no well documented study on the effect of calcium ions on efflux pumps. The ions of calcium are facilitated due to the hydroxide diffusion from the calcium hydroxide nanoparticles. The binding of the ions to the anionic moieties of the cell surface, neutralizes them and decreases the repulsion forces between them (Upadya et al., 2011). Therefore, it can be suggested that the nanoparticles of calcium act on the bacteria based on the ionic concentration of the same.
Platinum–Platinum is a precious metal that has been known to have adverse effects on many species of bacteria. Platinum nanoparticles have an affinity towards the protein subunits of the bacterial strains. They also have a preference to bind to the functional enzymes pertaining to replication and genetic material (Maye et al., 2005). The nanoparticles of platinum in colloidal forms have been reported to have
anti-inflammatory effects on lungs and skin edema (Yoshihisa et al., 2010). They cause less harm to the environment when compared with its other metal counterparts. Hence, they are the preferred class of nano- particles to mediate pathogens present in the natural environment (Rezaei-Zarchi et al., 2012). Lactobacillus species are found to be sus- ceptible to platinum nanoparticles, functionalized by sucrose. Even Pseudomonas stutzeri is treated effectively using the abovementioned combination of platinum. These nanoparticles have their antibacterial effects based on their sizes. Very small nanoparticles of platinum ranging from 1 to 3 nm exhibited good antibacterial activity whereas an increase in size made them compatible to bacteria. A slight increase of 4–21 nm also made the platinum nanoparticles lose their bactericidal activity against Pseudomonas aeruginosa (Gopal et al., 2013). The exclusive effects of platinum nanoparticles on efflux pump are still under experimental stages. There are no concrete data for the same. Mostly, focus has been given on the combinatorial effects of platinum nano- particles with structural building blocks of bacteria. Fig. 1 represents the graphical representation of the possible nanoparticles that aid in the deactivation of the efflux system.
Miscellaneous Nanoparticles - Aluminium nanoparticles were
tested against efflux-based MDR E. coli. The size of the particles was in the range of 10–100 nm. Their main mode of action was found to be cell wall disruption (Lee et al., 2019; Zaidi et al., 2017). Similarly, titanium was used against Staphylococcus aureus, Enterococcus faecalis, and E. coli. Oxidation of the intracellular components were found to be their mode of action (Hemeg, 2017; Lee et al., 2019; Rudramurthy et al., 2016). In polymeric nanoparticles, lignin has been successfully used against efflux-based MDR E. coli and found to harbour genes encoding for membrane proteins with an efflux function were upregulated, others down-regulated (Hasani et al., 2019; Slavin et al., 2021).
6. Proposed mechanism of action
The bacterial strains with lipopolysaccharide layers have better structural efficacy in preventing the antimicrobial activity of nano- particles. To be exact, the outer membrane is composed of porins as proteins, phosphate-associated lipids, and lipopolysaccharides (Couto et al., 2008). The other contemporary gram-positive bacteria has tei- choic acids in them (Gupta et al., 2014). Although their compositions vary, ultimately, they maintain the negative charge on their exterior (Zechini and Versace, 2009). Teichoic acid is a linear polymer made up of phosphate moieties. Along with phosphate, amines and carboxyl group also add up to the negative charge of the gram-positive strains (Chevalier et al., 2010). The lipid A composition of the lipopolysac- charide contributes to the negative charge of the gram-negative species (Bohnert and Kern, 2005). Lipid A is actually a carbohydrate that is phosphorylated. The core is essentially an oligosaccharide (German et al., 2008).
Bacteria that have adapted themselves to the changing environment
for survival pick up traits that help to survive—they stealth the drugs given against them. Even stimulus and disinfectants causing harm to the microbe are made ineffective by alterations made in the pathogen. This specialized population is denoted as persistent bacteria. The world bacterial population falling under this criterion is very less (around 1%). The very first resistant bacterial strain was reported in 1944. It was Staphylococcus aureus species (Imlay, 2013). Apart from creating trouble for the pharmaceutical industry to create new and advanced drugs on a regular basis, these species also have a key role in developing numerous long-time chronic conditions in patients (Imlay, 2015). The major mechanism by which nanoparticles tackle resistant bacteria is by creating stress. There is always a difference in the zeta potentials of the bacterium and the nanoparticles. Generally, bacteria will have around 26 mV, and the nanoparticles, for example, silver has around 15 mV. This difference in potential implies an attractive force between the pathogen and the nanoparticles. The outer envelope responds to the stress created by making the cell membrane of the bacteria positive. This
Fig. 1. The graphical representation of the possible nanoparticles that aid in the deactivation of the efflux system.
is done to neutralize the charge variations between the nanoparticles and the host. This host response tries to repel the nanoparticles away from the infectious agent. The host can perform this mechanism suc- cessfully if the nanoparticle concentration is kept below the inhibitory levels. If the nanoparticles are administered above the minimum levels, they can create enough charge difference to aggregate the nanoparticles on the surface of the bacteria. This leads to oxidative stress and inevi- table damage to the pathogen. When continuous low dosage levels are exposed to a particular pathogen, they act as a stimulus to enhance their survival for the future. Another mode of resistance is attained when the pathogen senses the presence of other species for synergistic growth feasibility. Naturally, Enterococcus faecalis is dormant in nature. It is found to be the main cause of infections in dental roots. They have also been seen in several resistant phenotypes (Lemire et al., 2013). When the habitat around the pathogen becomes unfavourable such as deficient in nutrients, they become dormant and cease to grow (Mijnendonckx et al., 2013). When co-species like Candida is sensed by Enterococcus faecalis, it gets reactivated and develops persistent pathways. The feasible ways for the bacteria to survive and adapt to unfavourable en- vironments consist of a passive mechanism and an active mode of defence. In the former mode, the cell does molecular modifications.
These changes can be in the guanosine penta phosphate, tetraphosphate,
modules of toxin and its counterpart, indole, etc. while the active mode of gaining resistance is through the efflux systems (Yamanaka et al., 2005). In this current review, the efflux system has been discussed; hence, the active mechanism of gaining persistence is given importance. Pu and team worked on the effect of efflux on the resistant strains and their cellular drug concentrations. They found that the fluorescently tagged antibiotics were found more in the external environment than inside of the cells. These results were very evident in single-cell micro- scopy. When these special species were sequenced and analysed, their efflux system has shown a group of over-expressive genes. Their rate of pumping the antibiotics out of the cell was also found to be in elevated levels when compared to their normal counterparts (Lee et al., 2010). Nanoparticles in their ionic forms are also pumped out effectively (Nallathamby et al., 2010). The size of the nanoparticles influences the efflux rates based on the species they are employed on.
The charge of the bacteria and the nanoparticles determines the
extent of the latter’s antibacterial activity. An experimental study was
performed on both gram-positive and negative strains having variations in charges. All the three charges of positive, neutral and negative were studied. The gram-positive strains under study were Staphylococcus aureus, Streptococcus mutans and Streptococcus pyogenes. Proteus vulgaris as well as E. coli were the gram negative bacteria studied. The results s were that the positively charged nanoparticles had the greatest affinity and heightened antibacterial responses when compared to the neutral and negative particles. When size-dependent studies were performed, the outcomes were still influenced by the surface charge than the dimension (Porras Go´mez et al., 2012). Along with oxidative stress mentioned in the previous section, osmotic pressure and pH (especially alkaline) also alter the surface charge of the microbe. Sigma factors that work for the extra-cytoplasmic functions lead to oxidative stress. They trigger the expression of many known and unknown genes of multiple functionalities. The most frequent function is to increase the production of lipopolysaccharide layers outside the cell. This affects the charge on the cell and its affinity towards nanoparticles. They also tend to intro- duce D-alanine amino acid in the membrane matrix to reduce the net negative charge. This step is again helpful for the pathogen to overcome cationic-based medications and therapies. Excessive external stimulus leads to the possibility of mutations. Mutations in the efflux system can elevate the bacterial threshold towards medications and treatments. The reactive oxygen species tend to up-regulate the genes associated with the efflux pumps and reduce the regulation of porins. This makes the bac- teria resistant to the specific drug (Tin et al., 2009).
7. Future aspects - species-specific efflux system
To overcome the efflux-based resistance pattern in bacteria, it is essential to focus on the molecular makeup of the pump. Each species has its own set of regulatory genes that the nanoparticles can target to retrieve the susceptibility of the species. A few of the most commonly found infectious pathogens are discussed below for their potential efflux carriers and genes. These genes can be used in the future as targets by the nanoparticles to make the pathogens susceptible to medications.
Gram-Negative – In this group of organisms, nanoparticles can be tailored to focus on RND in the outer membrane of the cell. This efflux system is the primary ejection pump that most of the gram-negative utilize to become persistent in the given environment.
E. coli - The efflux system has AcrAB carriers. Many persistent va- rieties are due to the mutation of the acrAB gene. They harbour resis- tance against fluoroquinolone, tigecycline, and cefuroxime. Many carry plasmid mediated efflux genes too. Tet B, C, and K genes have been found in plasmids of E. coli that harbor tetracycline resistance. These have been found to be working in flushing away angucyclinone antibi- otics. Several species attain Olaquindox resistance because of the OqxAB carrier pump. This whole process is TolC dependent. Various plasmids also have QepA/QepA2 genes to gain resistance against the conven- tional drugs (Cattoir et al., 2008; Hansen et al., 2004; K¨allman et al., 2003; Lautenbach et al., 2009).
Salmonella – they contain multiple efflux systems working simul- taneously to provide rapid resistance to drugs. Their basic efflux carriers associated would be AcrAB-TolC. Resistance have been successfully attained for ciprofloxacin, cyclohexane, fluoroquinolone, etc. 10% of the population are resistant because of the overexpression of AcrAB.
S. typhimurium has multiple genes activated for its efflux system. These genes are emr D, B, acrA, B, E, F, mdlB, mdtB, C and A. Mutations can lead to the deletion of TolC and AcrB carriers. When it comes to metal resistance, MdtABC and AcrD are found to be involved. Strains that harboured compromised TolC exhibited a heighted susceptibility. If the AcrAB-TolC carrier is altered, the overall expression of the pathogen is seen to be compromised (Braoudaki and Hilton, 2005; Chen et al., 2007; Nishino et al., 2006; Olliver et al., 2005; Piddock et al., 2000; Randall et al., 2001; Ricci et al., 2006). Some other pumps present in Salmonella species for providing MDR are MdfA, EmrAB, MdtK, etc.(Nishino et al., 2007).
Enterobacter – Apart from E. coli, the other most common entero- bacterium is Enterobacter aerogenes. AcrAB-TolC is responsible for MDR in these species. They have harboured resistance against clarithromycin, quinolones, chloramphenicol, erythromycin, tetracyclines, etc. Phenolic active compound resistance was feasible because of the presence of MarA carrier. The resistance to tigecycline is also mediated by the activation of RamA carrier A cryptic form of RND is found in Enterobacter species that is encoded by EefABC. These were found to be ineffective to provide persistence against ketolides and fluoroquinolones (Chollet et al., 2004; Masi et al., 2006).
Klebsiella – AcrAB is seen to be overexpressed in its homologous form. Mutations are generally reported in RamA and AcrR that often lead to the overexpression of efflux pumps. They play a pioneer role in the ß-lactam based resistance. EefABC has been reported to make Kleb- siella spp. tolerant to gastrointestinal acids. It is seen that the inactiva- tion of EefA, makes the bacteria susceptible again to several medications and therapy (Coudeyras et al., 2008; Schneiders et al., 2003).
Serratia – Serratia marcescens is one among the several naturally occurring resistant strains. They have 3 types of RND pumps SdeXY, SdeAB-HasF and SdeCDE. The analogue to TolC is HasF carrier. In plasmids, three types of genes encode the efflux pumps. These are SsmE, SmdAB and SmfY. In the natural habitat, TetA (41) is seen in
S. marcescens (Begic and Worobec, 2007; Matsuo et al., 2008; Stock et al., 2003).
Vibrio – they have 6 types of operons for the activation of efflux pumps. They have an RND-type pump. Among the 6, 2 are bile regu- lated, namely, VexCD and VexAB. These provide the bacterial resistance to bile. Hence, the bacteria survive well in the gut. VexEF confers to provide persistence against antibiotics. Many strains of vibrio have shown the presence of MATE pumps. Very few harbours ABC pumps. Efflux system is the major reason that Vibrio fluvialis is highly resistant to quinolone (Bina et al., 2006) ((Huda et al., 2003; Srinivasan et al., 2006).
Pseudomonas – these mostly contain soil bacteria. They have the tripartite system that works as an efflux system. Mex pumps are responsible for the survival of Pseudomonas aeruginosa. They are a type of RND pump. MexXY-OprM and MexAB-OprM are found to be over- expressed in several resistant varieties of Pseudomonas that cause blood infections (Driscoll et al., 2007; Strateva et al., 2007; Sugawara et al.,
2006). Novel systems have been reported recently for the same strain. They are TriABC-OpmH, MexMN-OprM, MexVW-OprM and MexP- QOpmE. MATE type pumps are also seen, which are denoted as PmpM (Zhou et al., 2006).
Acinetobacter - Among the different strains available Acinetobacter
baumannii has emerged as the most widely spread MDR strain in the world. RND has been reported for these species. AdeIJK is found to be the carrier involved in the process. Some additional genes reported are AdeXYZ and AdeDE. MATE, MFS and SMR are also documented for the same species (Bergogne-Berezin and Towner, 1996; Chu et al., 2006; Gilad and Carmeli, 2008; Su et al., 2005).
Gram Positive-these are the population of bacteria that are known to have peptidoglycan layers in their cell membranes. They seem to have lower resistance patterns than the gram-negative counterparts. A few well-known strains and their efflux mechanisms that can be addressed in future by nanotechnology and therapy, are discussed below.
Clostridium – these are gram positive strains that are found in various infections. They are anaerobic in nature. There are 4 main pathogen varieties of Clostridium that have shown an efflux pattern of resistance. These are Clostridium difficile, Clostridium tetani, Clostridium botulinum and Clostridium perfringens. Among the 4, Clostridium difficile has been shown to have the maximum hospital acquired resistance pattern. Most of the higher generation antibiotics used in hospitals tend to make these pathogenic strains resistant. It has been reported that cdeA is responsible for the MATE type efflux pump in Clostridium diffi- cile. These pumps are sodium ion coupled pumps. If this gene is found to be expressing in the plasmid DNA, then the resistance pattern is suc- cessfully conjugated to Clostridium perfringens. Cme is also found to be a gene responsible for efflux in Clostridium difficile. They are homologues of MefA/MefE. These are able to conjugate their resistant patterns to Enterococcus faecalis too. A mutation in the gyrB had s also reported tremendous resistance patterns that were not affected by the presence of efflux inhibitors. Tet (40) and Tet(P) are also found to be the part of the efflux expressing genes in Clostridium species (Kazimierczak et al., 2008; Owens et al., 2008).
Bacillus- It is found to have multiple pumps that aid the pathogen to
remove the toxic materials as well as antibiotics out of the system. A few common ones are Bmr3, Bmr and Blt. It has a fourth drug efflux system that gets expressed due to the mutations of the LmrB gene. Dimer shaped efflux is also found to be present in several Bacillus subtilis species. This is represented by Tet(L), which is a tetracycline efflux system. An ABC transporter has been discovered in Bacillus subtilis species that is func- tional in nature and is denoted as YvcC (BmrA) (Bast et al., 2004; Ohki and Tateno, 2004).
Listeria monocytogenes – they are pathogens found in contaminated foods. They have been susceptible to many medications for years. Nevertheless, recently, these strains have started harbouring some form of resistance. They have tet(K), floR and tet(A) as efflux carriers on their membranes. MdrL is found to be a partial transporter that can cause resistance patterns in these strains (Ramaswamy et al., 2007; Soumet et al., 2005).
Staphylococcus – Efflux is a very important technique opted by
Staphylococcus aureus to thrive against different medications. It has separate genes in the chromosomes and the plasmid to express and activate their efflux systems. In the chromosome, NorA genes are found. In the plasmid, QacA/B and MsrA are found. Few more additional genes encoding for pumps are Tet (38), MdeA, NorB, MepA, AbcA. SepA, NorC and SdrM. They have almost all types of efflux carriers available to help the organism with the ejection of drugs from its internal compartments. In cases of abscess, NorB is seen to overexpress the pathogen to make it highly tolerable to medications (Ding et al., 2008; Hassan et al., 2007; Truong-Bolduc and Hooper, 2007).
Enterococcus faecalis - Enterococcus faecalis is another gram-positive
strain that is very much resistant to several antibiotic drugs. Its r mode of efflux-based resistance is an ABC transporter. EfrAB is the gene associ- ated with it. Lsa genes are also associated with potential resistance
patterns in Enterococcus faecalis. Few drugs such as norfloxacin tend to instil mutations in bacteria that have non-EmeA strain, which is similar to NorA pump. Majority of them will contain msrC. This gene encodes for the elimination of erythromycin (Aslangul et al., 2006; Lee et al., 2003).
Streptococcus pneumoniae – this pathogen is very well known to
cause various pneumococcal related illnesses. Efflux is exhibited in most of the Streptococcus species. Its efflux is generally of MFS type. These are denoted as PmrA, MefA and MefE. PatA and B are also suggested to be a part of the ciprofloxacin resistance in Streptococcus species. These are a type of ABC transporter proteins. A similar type of gene i. e SP2073 to 75 is also noticed in the strain that regulates the ejection of some quino- lones. . If the inactivation of other carriers for efflux such as MATE and MFS is initiated, Streptococcus doesn’t show much change in the sus- ceptibility trend. Mef(A) gene is also reported to be the causative agent for the presence of efflux mechanism in M phenotype Streptococcus species (Cousin et al., 2003; Jumbe et al., 2006; Marrer et al., 2006). Table 3 depicts the nanoparticles used till date to treat potential infec- tious pathogens that use efflux systems for their survival.
Size seems to play a major role when it comes to efflux hampering by
metallic nanoparticles. Smaller particles reside longer within the path- ogenic cell when compared to their larger counterparts. Hence mani- festing size influenced efflux kinetics of the membrane pumps (Banerjee et al., 2010). In P. aeruginosa, it was reported that a single nanoparticle tends to have a higher accumulation in the internal components of a wild-type strain when compared to MexAB-OprM overexpressed strains. While further investigating, it was found that the residual time of the nanoparticles increase (up to 10 times) when given in combination with an efflux inhibitor like Carbonyl Cyanide m-Chlorophenylhydrazine (CCCP) or any form of medication (Nallathamby et al., 2010). When the drug concentration is administered in optimal amounts in conjugation with nanoparticles, the efficacy has been quite remarkable. The efflux pumps extrude aztreonam when administered in lower quantities (0–3.13 µg/mL). At optimal concentrations (31.3 µg/mL), the drug alters the cell membrane to the extent that the accumulation of the nano- particle becomes 8-fold, hence killing the pathogen. A similar effect has been seen between chloramphenicol, amoxicillin, clindamycin, eryth- romycin, penicillin G, vancomycin, and silver nanoparticles (Nalla- thamby et al., 2010; Okkeh et al., 2021). Thus, its not only the nanoparticle that aids the drug but vice versa is also seen in many strains.
The overall bactericidal activity of the antibiotic is enhanced when used in combination with nanoparticles. In 2007, Saha and team streptomycin, kanamycin or ampicillin conjugated gold nanoparticles remarkably reduced the minimum inhibitory concentration against a broad spectrum of pathogens (Saha et al., 2007). Similarly, in 2017, gold nanoparticles with fluoroquinolones worked very efficiently against MDR E. coli (Gupta et al., 2017). The unique structure of the nano- particles gives an advantage over commercial drugs in terms of anti- biotic resistance (Zazo et al., 2016). When tailored and packed with various medications, resistance is unlikely to occur in a pathogen as spontaneous and consecutive mutations would be required by the microbe to survive (Zhao and Jiang, 2013). The efficiency of silver nanoparticles with antibiotics against beta-lactamase E. coli, Staphylo- coccus aureus, Pseudomonas aeruginosa, etc., is quite effective in lower concentrations (Scandorieiro et al., 2016). The combination of antibi- otics with nanoparticles seems to reverse the resistance in a pathogen and increases the efficiency of many drugs like clindamycin, ceftazi- dime, erythromycin, ciprofloxacin, vancomycin, polymyxin B, ampi- cillin, methicillin, etc. (Hemeg, 2017). But these studies have been reported mostly in lab isolates or animal models. Translations of the same in human clinical trials have not yielded much clinically signifi- cant changes in the overall metabolic, urine and haematological profile
of the test subject. Healthy volunteers were administered with 10 ppm
and 32 ppm of silver nanoparticles ranging from sizes 10 nm to 40 nm respectively. This brings into attention the need for optimization of
nanoparticle dosage in humans (Munger et al., 2014).
8. Limitations and future perspectives
Limitations involving the utilization of nanomaterials as a potential remedy to tackle efflux pump-based resistance are that these particles themselves harbor unpredictable hazardous traits to the biota around them. Continuous exposure of the microbial population to high con- centrations of nanomaterials can lead to the attainment of another un- desired trait by the pathogen, which needs to be addressed later. The best perspective that could be followed while working with nano- materials for efflux impediments would be tailoring the nanoparticles very specific to the pump and protein associated with it. This would lead to very lower dosages of nanoparticles yielding remarkable outcomes in making these potential pathogens susceptible to lower generations of antibiotics again. The genes expressing the pumps can be specifically targeted by the designed nanoparticles to solve the multiple drug resistance issue at a very primary level. The therapeutic ability of nanomaterials is quite limited to its clearance, mode of action, nature of the target, dosage, physicochemical, morphological, chemical proper- ties, the solvent used, environmental factors, and toxicity generated against the pathogens (Beyth et al., 2015). Very specific and optimal combinations of nanomaterials with parent antibiotics can avoid the emergence of drug resistance. But the translation of this experimental hypothesis into clinical evidence requires detailed perception with
proper implementation of pharmacodynamics and pharmacokinetic
studies. It has been reported previously that there are a synergistic effect between antibiotics conjugated nanomaterials, especially metallic nanoparticles (Baptista et al., 2018; Pelgrift and Friedman, 2013). Among the various features opted by a nanoparticle against microor- ganisms, controlling the efflux system is one of them. The large aspect ratio of the materials increases their contact with the pathogen hence deactivate the efflux system in many ways (Dura´n et al., 2016; Hemeg, 2017).
Currently nanoparticles are effectively used as surface coatings and dermal therapeutics. Scale-up and production guidelines for manufacturing nanoparticle and antibiotic conjugations need to be laid with efficient standardization of toxicology assays and protocols. Efforts are to be taken for optimizing the drug dosage for oral administration in humans so that the drug nanoparticle combination can reach the phar- maceutical market and cease the rise of MDR (Zaidi et al., 2017). Lastly, the economic impact of the clinical translation of this combination must be addressed in terms of efficiency in humans (Zazo et al., 2016).
9. Conclusion
The current review article focuses on the effects of nanoparticles on efflux systems. Many nanoparticles have shown promising results in labs and bench-scale studies. Their outcomes have been so remarkable that several patents are also filed to scale them up for commercial applica- tions. However, when it comes to the clinical implementation of these nanoparticles, there are numerous challenges that need to be tackled. Even if the clinical translation of the particles becomes a success, the risk assessment, especially, time-based assessment is always an unanswered question. Assessment of viable cells and systems varies from the models used. It is always essential to know about the various modes these par- ticles opt to imply their antibacterial activity. Their physical dimensions offer many distinctive advantages to various ineffective drugs. They have been successfully conjugated with medications used in all areas of the body from dermal to gastrointestinal. The main efflux carrier found to have a primary responsibility to provide resistance is the RND carrier system. Secondly, MATE and ABC carriers with mutations also result in persistent bacterial species. The major mechanisms of their activities have been discussed in the present article. They mainly focused on the disruption of the cell wall or damaging the subunits of the carrier sys- tem. Very little evidence is there for the manipulation of genetic makeup
Table 3
Different Nanoparticles used to treat Efflux based potential infectious pathogens.
Category Nanoparticle Organism Mean Shape Average Size/ Concentration range
Average MIC value
Mechanism References
Polymer Chitosan E. coli and P. aeruginosa N/A Below 500 nm – Heightened affinity to the inhibitor
MexEF-OprN overexpression inhibited
(Helander et al., 2001; Lee et al., 2019; Pelgrift and Friedman, 2013; Tin et al., 2009; Usman et al., 2013; Yang et al., 2021)
Lignin with silver
S. aureus, S. epidermidis, P. aeruginosa, K. pneumoniae, and
A. baumannii
shells 42 nm 5–25 µg/mL Genes encoding for membrane proteins
with an efflux function were upregulated, others down regulated
Slavin et al. (2021)
Non metal Nitric oxide K. pneumoniae,
S. aureus, E. faecalis, E. coli,
P. aeruginosa
N/A 1.25–5 mM – Altered integrity of the plasma barrier (HuhandY and Kwon, 2011; Lee et al., 2019; Raafat
et al., 2008)
Low-cost metal Zinc A. baumannii Rod, spheres 10–40 nm 20 µg/mL breakdown of the membrane integrity
and generation of reactive oxygen species efflux system (NorA) falls short of energy
(Banoee et al., 2010; Couto et al., 2008; Ghasemi and Jalal, 2016; Lee et al., 2019; Venubabu Thati et al., 2010; Yang et al., 2021)
Magnesium S. aureus or B. subtilis N/A Average 100 nm – generate reactive species of oxygen (Hajipour et al., 2013; Lee et al., 2019; Pelgrift and
Friedman, 2013)
Copper S. aureus, E. faecalis and E. coli Spherical, quasi
spherical, rod, rectangle
Less than 300 nm E. coli- 20 µg/
mL S. aureus was 140 µg/mL
stop production of biofilms and enhance sensitivity
(Bagchi et al., 2013; Christena et al., 2015; Ruparelia et al., 2008; Yang et al., 2021)
Iron M. smegmatis N/A 1–100 nm – oxidation of the intracellular
components
(Lee et al., 2008, 2019; Sies, 1997; Xing et al., 2013)
Aluminium E. coli N/A 10–100 nm – Cell wall disruption (Lee et al., 2019; Zaidi et al., 2017)
Mineral based Nanoparticles
Titanium S. aureus, E. faecalis and E. coli N/A 30–45 nm – oxidation of the intracellular
components
Calcium S. mutans and E. faecalis spheres – – The binding of the ions to the anionic
moieties of the cell surface, neutralize them and decreases the repulsion forces between them
(Hemeg, 2017; Lee et al., 2019; Rudramurthy et al., 2016)
(Drummond, 2008; Lee et al., 2019; Lim et al., 2002)
Quartz
Nanoparticle
Silica S. aureus crystals Less than 400 nm – Cell wall disruption (Lee et al., 2019; Zaidi et al., 2017)
Noble metals Platinum P. stutzeri, P. aeruginosa,
Lactobacillus sp.
Spheres 1–3 nm
4–21 nm
5 µg/mL Exhibited good antibacterial activity,
mode for efflux deactivation yet to be investigated
(Gopal et al., 2013; Lee et al., 2019; Rezaei-Zarchi
et al., 2012; Yang et al., 2021; Yoshihisa et al., 2010)
Silver P. aeruginosa,
B. subtilis,
S. aureus,
S. typhi luteus, Micrococcus sp,
Spherical, rod, polyhedron
Less than 100 nm 33 nM MexAB-OprM overexpression inhibited
Over accumulation of the nanoparticle leads to condensation pressure on the chromosomes
(Bera et al., 2014; Kim et al., 2007; Kyriacou et al., 2004; Lee et al., 2019; Li et al., 2005; Xu et al., 2004; Yang et al., 2021)
Gold Enterococcus sp. E. coli resistant to vancomycin, Pseudomonas species resistant to methicillin Corynebacterium
Spheres, nanorod Less than 100 nm 5 µg/mL accumulate in the intracellular space
damage to the efflux pump
(Bahrami et al., 2014; Gu et al., 2003; Hayden et al., 2012; Hyland et al., 2006; Khan et al., 2016; Lee et al., 2019; Mohamed et al., 2017; Okkeh et al., 2021; Rosi et al., 2006; Yang et al., 2021; Zhao et al., 2010)
in the efflux system. The present review has provided the compiled data about the possible genes targeted using nanoparticles to aid the current antibiotics to function against many resistant species. Novel efforts are underway to incorporate these particles in medical devices and equip- ment to avoid microbial growth and proliferation. However, systemic administration of nanoparticles is still not accepted to a great extent. Proper guidelines are yet to be devised logically and rationally to opti- mize and form these nanoparticles against MDR species. These norms should cover the whole aspect and the process involved in the making of drug nanoparticles. The modes of characterization, the properties to be considered, the steps and features for biocompatibility and norms for data comparison and acquisition in both lab and clinical studies should be addressed well in the future. Suppose nanoparticles are made target specific to the carriers of efflux and genes for the respective species. In that case, the conventional medications will have better efficacy in treating patients worldwide. The load on generating new and novel drugs will reduce, and the cost of treatment will come down as older medications would be put to use again. The study on the targeting of the nanoparticles towards these specific genes and efflux carriers should be of utmost preference in medical microbiology in the upcoming years.
Author statement
Nibedita Dey: Conceptualization, Methodology, Writing – original draft. Kamatchi C: Writing – original draft. Vickram A S: Writing- Reviewing and Editing. Anbarasu K: Writing-Reviewing and Editing, Thanigaivel S: Writing-Reviewing and Editing, Jeyanthi Palanivelu: Writing-Reviewing and Editing, Arivalagan Pugazhendhi: Writing- Reviewing and Editing, Vinoth Kumar Ponnusamy: Supervision, Proj- ect administration.
Declaration of competing interest
The authors declare that they have no known competing financial interests or personal relationships that could have appeared to influence the work reported in this paper.
Acknowledgments
Authors thanks for the grant support by Saveetha Institute of Medical and Technical Sciences, Chennai, India. This work was supported financially by the grant MOST 110-2113-M-037-009- from Ministry of Science and Technology, Taiwan, and also funded by the Research Center for Environmental Medicine, Kaohsiung Medical University, Kaohsiung, Taiwan from “The Featured Areas Research Center Program within the framework of the Higher Education Sprout Project” by the Ministry of Education (MOE) in Taiwan.
References
Ashajyothi, C., et al., 2016. Antibiofilm activity of biogenic copper and zinc oxide nanoparticles-antimicrobials collegiate against multiple drug resistant bacteria: a nanoscale approach. J. Nanostructure Chem. 6, 329–341.
Aslangul, E., et al., 2006. Acquired gentamicin resistance by permeability impairment in Enterococcus faecalis. Antimicrob.Agents Chemotherapy 50, 3615–3621.
Ayukekbong, J.A., et al., 2017. The threat of antimicrobial resistance in developing countries: causes and control strategies. Antimicrob. Resist. Infect. Contr. 6, 1–8.
Badar, W., Khan, M.A.U., 2020. Analytical study of biosynthesised silver nanoparticles against multi-drug resistant biofilm-forming pathogens. IET Nanobiotechnol. 14, 331–340.
Bagchi, B., et al., 2013. In situ synthesis and antibacterial activity of copper nanoparticle loaded natural montmorillonite clay based on contact inhibition and ion release. Colloids Surf. B Biointerfaces 108, 358–365.
Bahrami, K., et al., 2014. Hydroxyl capped silver-gold alloy nanoparticles: characterization and their combination effect with different antibiotics against Staphylococcus aureus. Nanomedicine Journal 1, 155–161.
Banerjee, M., et al., 2010. Heightened reactive oxygen species generation in the antimicrobial activity of a three component iodinated chitosan silver nanoparticle composite. Langmuir 26, 5901–5908.
Banigan, J.R., et al., 2015. A structured loop modulates coupling between the substrate- binding and dimerization domains in the multidrug resistance transporter EmrE.
J. Biol. Chem. 290, 805–814.
Banoee, M., et al., 2010. ZnO nanoparticles enhanced antibacterial activity of ciprofloxacin against Staphylococcus aureus and Escherichia coli. J. Biomed. Mater. Res. B Appl. Biomater. 93, 557–561.
Baptista, P.V., et al., 2018. Nano-strategies to fight multidrug resistant bacteria—“A Battle of the Titans”. Front. Microbiol. 9, 1441.
Bast, D.J., et al., 2004. Type II topoisomerase mutations in Bacillus anthracis associated with high-level fluoroquinolone resistance. J. Antimicrob. Chemother. 54, 90–94.
Bay, D.C., et al., 2008. Small multidrug resistance proteins: a multidrug transporter family that continues to grow. Biochim. Biophys. Acta Biomembr. 1778, 1814–1838.
Begic, S., Worobec, E.A., 2007. Fluoroquinolone resistance of Serratia marcescens: sucrose, salicylate, temperature, and pH induction of phenotypic resistance. Can. J. Microbiol. 53, 1239–1245.
Beketskaia, M.S., et al., 2014. Outer membrane protein OmpW participates with small multidrug resistance protein member EmrE in quaternary cationic compound efflux. J. Bacteriol. 196, 1908–1914.
Bera, R., et al., 2014. Antimicrobial activity of fluorescent A g nanoparticles. Lett. Appl.
Microbiol. 58, 520–526.
Bergogne-Berezin, E., Towner, K., 1996. Acinetobacter spp. as nosocomial pathogens: microbiological, clinical, and epidemiological features. Clin. Microbiol. Rev. 9, 148.
Beyth, N., et al., 2015. Alternative antimicrobial approach: nano-antimicrobial materials. Evid. base Compl. Alternative Med. https://doi.org/10.1155/2015/246012, 2015.
Bina, J.E., et al., 2006. Characterization of the Vibrio cholerae vexAB and vexCD efflux systems. Arch. Microbiol. 186, 171–181.
Bohnert, J.A., Kern, W.V., 2005. Selected arylpiperazines are capable of reversing multidrug resistance in Escherichia coli overexpressing RND efflux pumps.
Antimicrob.Agents Chemotherapy 49, 849–852.
Boucher, H.W., et al., 2009. Bad bugs, no drugs: no ESKAPE! an update from the Infectious Diseases Society of America. Clin. Infect. Dis. 48, 1–12.
Braoudaki, M., Hilton, A.C., 2005. Mechanisms of resistance in Salmonella enterica adapted to erythromycin, benzalkonium chloride and triclosan. Int. J. Antimicrob. Agents 25, 31–37.
Brown, A.N., et al., 2012. Nanoparticles functionalized with ampicillin destroy multiple- antibiotic-resistant isolates of Pseudomonas aeruginosa and Enterobacter aerogenes and methicillin-resistant Staphylococcus aureus. Appl. Environ. Microbiol. 78, 2768–2774.
Bystro¨m, A., et al., 1985. The antibacterial effect of camphorated paramonochlorophenol, camphorated phenol and calcium hydroxide in the treatment of infected root canals. Dent. Traumatol. 1, 170–175.
Carmen Chifiriuc, M., et al., 2014. Contribution of antimicrobial peptides to the development of new and efficient antimicrobial strategies. Curr. Proteonomics 11, 98–107.
Cattoir, V., et al., 2008. Plasmid-mediated quinolone resistance pump QepA2 in an Escherichia coli isolate from France. Antimicrob.Agents Chemotherapy 52, 3801–3804.
Chatterjee, A.K., et al., 2014. Mechanism of antibacterial activity of copper nanoparticles. Nanotechnology 25, 135101.
Chaves, T.P., et al., 2015. Antibiotic resistance modulation by natural products obtained from Nasutitermes corniger (Motschulsky, 1855) and its nest. Saudi J. Biol. Sci. 22, 404–408.
Chen, S., et al., 2007. Contribution of target gene mutations and efflux to decreased susceptibility of Salmonella enterica serovar Typhimurium to fluoroquinolones and other antimicrobials. Antimicrob.Agents Chemotherapy 51, 535–542.
Cheng, L., et al., 2012. Antibacterial amorphous calcium phosphate nanocomposites with a quaternary ammonium dimethacrylate and silver nanoparticles. Dent. Mater. 28, 561–572.
Chertok, B., et al., 2008. Iron oxide nanoparticles as a drug delivery vehicle for MRI monitored magnetic targeting of brain tumors. Biomaterials 29, 487–496.
Chevalier, J., et al., 2010. Quinazoline derivatives are efficient chemosensitizers of antibiotic activity in Enterobacter aerogenes, Klebsiella pneumoniae and Pseudomonas aeruginosa resistant strains. Int. J. Antimicrob. Agents 36, 164–168.
Choi, O., , et al.Jr, L., Surampalli, R., 2008. Z. Hu. Water Res. 42, 3066–3074.
Chollet, R., et al., 2004. The AcrAB-TolC pump is involved in macrolide resistance but not in telithromycin efflux in Enterobacter aerogenes and Escherichia coli.
Antimicrob.Agents Chemotherapy 48, 3621–3624.
Christena, L.R., et al., 2015. Copper nanoparticles as an efflux pump inhibitor to tackle drug resistant bacteria. RSC Adv. 5, 12899–12909.
Chu, Y.W., et al., 2006. Presence of active efflux systems AdeABC, AdeDE and AdeXYZ in different Acinetobacter genomic DNA groups. J. Med. Microbiol. 55, 477–478.
Coudeyras, S., et al., 2008. A tripartite efflux pump involved in gastrointestinal colonization by Klebsiella pneumoniae confers a tolerance response to inorganic acid. Infect. Immun. 76, 4633–4641.
Cousin Jr., S.L., et al., 2003. Acquired macrolide resistance genes and the 1 bp deletion in the mtrR promoter in Neisseria gonorrhoeae. J. Antimicrob. Chemother. 51, 131–133.
Coutinho, H.D., et al., 2009. Termite usage associated with antibiotic therapy: enhancement of aminoglycoside antibiotic activity by natural products of Nasutitermes corniger (Motschulsky 1855). BMC Compl. Alternative Med. 9, 1–4.
Couto, I., et al., 2008. Efflux-mediated response of Staphylococcus aureus exposed to ethidium bromide. J. antimicrobial chemotherapy 62, 504–513.
Davidson, A.L., et al., 2008. Structure, function, and evolution of bacterial ATP-binding cassette systems. Microbiol. Mol. Biol. Rev. 72, 317–364.
Dibrov, P., et al., 2002. Chemiosmotic mechanism of antimicrobial activity of Ag in Vibrio cholerae. Antimicrob.Agents Chemotherapy 46, 2668–2670.
Ding, Y., et al., 2008. NorB, an efflux pump in Staphylococcus aureus strain MW2, contributes to bacterial fitness in abscesses. J. Bacteriol. 190, 7123–7129.
Distel, J.W., et al., 2002. Biofilm formation in medicated root canals. J. Endod. 28, 689–693.
Driscoll, J.A., et al., 2007. The epidemiology, pathogenesis and treatment of Pseudomonas aeruginosa infections. Drugs 67, 351–368.
Drummond, J.L., 2008. Degradation, fatigue, and failure of resin dental composite materials. J. Dent. Res. 87, 710–719.
Dur´an, N., et al., 2016. Silver nanoparticles: a new view on mechanistic aspects on antimicrobial activity. Nanomed. Nanotechnol. Biol. Med. 12, 789–799.
Duval, M., et al., 2018. HflXr mediates a novel antibiotic resistance mechanism 1 2. Proc.
Natl. Acad. Sci. U.S.A. 115, 13359–13364.
Fayaz, A.M., et al., 2010. Biogenic synthesis of silver nanoparticles and their synergistic effect with antibiotics: a study against gram-positive and gram-negative bacteria. Nanomed. Nanotechnol. Biol. Med. 6, 103–109.
German, N., et al., 2008. Synthesis and evaluation of PSSRI-based inhibitors of Staphylococcus aureus multidrug efflux pumps. Bioorg. Med. Chem. Lett 18, 1368–1373.
Ghasemi, F., Jalal, R., 2016. Antimicrobial action of zinc oxide nanoparticles in combination with ciprofloxacin and ceftazidime against multidrug-resistant Acinetobacter baumannii. J. global antimicrobial resistance 6, 118–122.
Gilad, J., Carmeli, Y., 2008. Treatment options for multidrug-resistant Acinetobacter species. Drugs 68, 165–189.
Gonza´lez-Bello, C., 2017. Antibiotic adjuvants–A strategy to unlock bacterial resistance to antibiotics. Bioorg. Med. Chem. Lett 27, 4221–4228.
Gopal, J., et al., 2013. Bacterial toxicity/compatibility of platinum nanospheres, nanocuboids and nanoflowers. Sci. Rep. 3, 1–8.
Gu, H., et al., 2003. Using biofunctional magnetic nanoparticles to capture vancomycin- resistant enterococci and other gram-positive bacteria at ultralow concentration.
J. Am. Chem. Soc. 125, 15702–15703.
Gupta, A., et al., 2017. Synergistic antimicrobial therapy using nanoparticles and antibiotics for the treatment of multidrug-resistant bacterial infection. Nano Futures 1, 015004.
Gupta, R.P., et al., 2011. The Multidrug Transporter Pdr5: a Molecular Diode?. Gupta, S., et al., 2014. Efflux inhibition with verapamil potentiates bedaquiline. In:
Mycobacterium tuberculosis. Antimicrobial Agents and Chemotherapy, vol. 58, pp. 574–576.
Gurunathan, S., et al., 2014. Enhanced antibacterial and anti-biofilm activities of silver nanoparticles against Gram-negative and Gram-positive bacteria. Nanoscale research letters 9, 1–17.
Hajipour, M.J., et al., 2013. Erratum: antibacterial properties of nanoparticles:[trends in biotechnology 30 (2012). Trends Biotechnol. 31, 499–511, 61-62.
Hammami, R., Fliss, I., 2010. Current trends in antimicrobial agent research: chemo-and bioinformatics approaches. Drug Discov. Today 15, 540–546.
Hancock, R.E., 1997. The bacterial outer membrane as a drug barrier. Trends Microbiol.
5, 37–42.
Hansen, L.H., et al., 2004. Plasmid-encoded multidrug efflux pump conferring resistance to olaquindox in Escherichia coli. Antimicrob.Agents Chemotherapy 48, 3332–3337.
Hao, Z., et al., 2014. The multiple antibiotic resistance regulator MarR is a copper sensor in Escherichia coli. Nat. Chem. Biol. 10, 21–28.
Hasani, A., et al., 2019. Metal nanoparticles and consequences on multi-drug resistant bacteria: reviving their role. SN Applied Sciences 1, 1–13.
Hassan, K.A., et al., 2015a. Homologs of the Acinetobacter baumannii AceI transporter represent a new family of bacterial multidrug efflux systems. mBio 6, e01982, 14.
Hassan, K.A., et al., 2015b. Homologs of the Acinetobacter baumannii AceI transporter represent a new family of bacterial multidrug efflux systems. mBio 6.
Hassan, K.A., et al., 2007. Active export proteins mediating drug resistance in staphylococci. J. Mol. Microbiol. Biotechnol. 12, 180–196.
Hayden, S.C., et al., 2012. Aggregation and interaction of cationic nanoparticles on bacterial surfaces. J. Am. Chem. Soc. 134, 6920–6923.
Helander, I., et al., 2001. Chitosan disrupts the barrier properties of the outer membrane of Gram-negative bacteria. Int. J. Food Microbiol. 71, 235–244.
Hemeg, H.A., 2017. Nanomaterials for alternative antibacterial therapy. Int. J. Nanomed.
12, 8211.
Huda, M.N., et al., 2003. Gene cloning and characterization of VcrM, a Na -coupled multidrug efflux pump, from Vibrio cholerae non-O1. Microbiol. Immunol. 47, 419–427.
HuhandY, A., Kwon, J., 2011. Nanoantibiotics’: anewparadigmfortreatinginfectiousdis- easesusingnanomaterialsintheantibioticsresistantera. JournalofControlledRelease 156, 128–145.
Hyland, R.M., et al., 2006. N-acetyllactosamine conjugated to gold nanoparticles inhibits enteropathogenic Escherichia coli colonization of the epithelium in human intestinal biopsy specimens. Infect. Immun. 74, 5419–5421.
Imlay, J.A., 2015. Diagnosing oxidative stress in bacteria: not as easy as you might think.
Curr. Opin. Microbiol. 24, 124–131.
Ismail, B., et al., 2018. Predictors of polymyxin B treatment failure in Gram-negative healthcare-associated infections among critically ill patients. J. Microbiol. Immunol. Infect. 51, 763–769.
Israil, A., Chifiriuc, M., 2009. Bacterial Communication: New Concepts in the Antimicrobial Therapy. Asclepius House.
Jumbe, N.L., et al., 2006. Quinolone efflux pumps play a central role in emergence of fluoroquinolone resistance in Streptococcus pneumoniae. Antimicrob.Agents Chemotherapy 50, 310–317.
Kaatz, G.W., et al., 2005. Multidrug resistance in Staphylococcus aureus due to overexpression of a novel multidrug and toxin extrusion (MATE) transport protein. Antimicrob.Agents Chemotherapy 49, 1857–1864.
K¨allman, O., et al., 2003. Contribution of efflux to cefuroxime resistance in clinical isolates of Escherichia coli. Scand. J. Infect. Dis. 35, 464–470.
Kaplan, H.B., Greenberg, E., 1985. Diffusion of autoinducer is involved in regulation of the Vibrio fischeri luminescence system. J. Bacteriol. 163, 1210–1214.
Kazimierczak, K.A., et al., 2008. A new tetracycline efflux gene, tet (40), is located in tandem with tet (O/32/O) in a human gut firmicute bacterium and in metagenomic library clones. Antimicrob.Agents Chemotherapy 52, 4001–4009.
Khameneh, B., et al., 2016. Breakthroughs in bacterial resistance mechanisms and the potential ways to combat them. Microb. Pathog. 95, 32–42.
Khan, M.S., et al., 2016. Highly efficient gold nanorods assisted laser phototherapy for rapid treatment on mice wound infected by pathogenic bacteria. J. Ind. Eng. Chem. 36, 49–58.
Kim, J.S., et al., 2007. Antimicrobial effects of silver nanoparticles. Nanomed.
Nanotechnol. Biol. Med. 3, 95–101.
Kulshrestha, S., et al., 2016. Calcium fluoride nanoparticles induced suppression of Streptococcus mutans biofilm: an in vitro and in vivo approach. Appl. Microbiol. Biotechnol. 100, 1901–1914.
Kulshrestha, S., et al., 2017. Antibiofilm efficacy of green synthesized graphene oxide- silver nanocomposite using Lagerstroemia speciosa floral extract: a comparative study on inhibition of gram-positive and gram-negative biofilms. Microb. Pathog. 103, 167–177.
Kumar, M.N.R., 2000. A review of chitin and chitosan applications. React. Funct. Polym.
46, 1–27.
Kumar, S., Varela, M.F., 2013. Molecular mechanisms of bacterial resistance to antimicrobial agents. Chemotherapy 14, 522–534.
Kuroda, T., Tsuchiya, T., 2009. Multidrug efflux transporters in the MATE family.
Biochim. Biophys. Acta Protein Proteonomics 1794, 763–768.
Kyriacou, S.V., et al., 2004. Using nanoparticle optics assay for direct observation of the function of antimicrobial agents in single live bacterial cells. Biochemistry 43, 140–147.
Lambert, P.A., 2005. Bacterial resistance to antibiotics: modified target sites. Adv. Drug Deliv. Rev. 57, 1471–1485.
Lautenbach, E., et al., 2009. Gastrointestinal tract colonization with fluoroquinolone- resistant Escherichia coli in hospitalized patients: changes over time in risk factors for resistance. Infect. Contr. Hosp. Epidemiol.: the official journal of the Society of Hospital Epidemiologists of America 30, 18.
Lee, C., et al., 2008. Bactericidal effect of zero-valent iron nanoparticles on Escherichia coli. Environ. Sci. Technol. 42, 4927–4933.
Lee, E.-W., et al., 2003. EfrAB, an ABC multidrug efflux pump in Enterococcus faecalis.
Antimicrob.Agents Chemotherapy 47, 3733–3738.
Lee, K.J., et al., 2010. Probing of multidrug ABC membrane transporters of single living cells using single plasmonic nanoparticle optical probes. Anal. Bioanal. Chem. 397, 3317–3328.
Lee, M.D., et al., 2001. Microbial fermentation-derived inhibitors of efflux-pump- mediated drug resistance. Il Farmaco 56, 81–85.
Lee, N.-Y., et al., 2019. Nanoparticles in the treatment of infections caused by multidrug- resistant organisms. Front. Pharmacol. 10, 1153.
Lemire, J., et al., 2013. Box 3: the Fenton reaction, free radical chemistry and metal poisoning. Nat. Rev. Microbiol. 11, 371–384.
Lewinski, N., et al., 2008. Cytotoxicity of nanoparticles. Small 4, 26–49.
Li, P., et al., 2005. Synergistic antibacterial effects of ß-lactam antibiotic combined with silver nanoparticles. Nanotechnology 16, 1912.
Li, W.-R., et al., 2011. Antibacterial effect of silver nanoparticles on Staphylococcus aureus. Biometals 24, 135–141.
Lim, B.-S., et al., 2002. Reduction of polymerization contraction stress for dental composites by two-step light-activation. Dent. Mater. 18, 436–444.
Liu, J., et al., 2013. Genetic variations in the active efflux pump genes acrA/B and tolC in different drug-induced strains of Escherichia coli CVCC 1547. Genet. Mol. Res. 12, 2829–2836.
Lok, C., , et al.Sun, H.z., 2007. PKH tam, JF chiu and CM che. J. Biol. Inorg. Chem. 12, 527–534.
Magiorakos, A.-P., et al., 2012. Multidrug-resistant, extensively drug-resistant and pandrug-resistant bacteria: an international expert proposal for interim standard definitions for acquired resistance. Clinical microbiology and infection 18, 268–281.
Maillard, J.-Y., Hartemann, P., 2013. Silver as an antimicrobial: facts and gaps in knowledge. Crit. Rev. Microbiol. 39, 373–383.
Marrer, E., et al., 2006. Global transcriptome analysis of the responses of a fluoroquinolone-resistant Streptococcus pneumoniae mutant and its parent to ciprofloxacin. Antimicrob.Agents Chemotherapy 50, 269–278.
Masi, M., et al., 2006. Production of the cryptic EefABC efflux pump in Enterobacter aerogenes chloramphenicol-resistant mutants. J. Antimicrob. Chemother. 57, 1223–1226.
Masi, M., et al., 2017. Mechanisms of envelope permeability and antibiotic influx and efflux in Gram-negative bacteria. Nature microbiology 2, 1–7.
Matsuo, T., et al., 2008. SmdAB, a heterodimeric ABC-type multidrug efflux pump, in Serratia marcescens. J. Bacteriol. 190, 648–654.
Maye, M.M., et al., 2005. Mediator template assembly of nanoparticles. J. Am. Chem.
Soc. 127, 1519–1529.
Mijnendonckx, K., et al., 2013. Antimicrobial silver: uses, toxicity and potential for resistance. Biometals 26, 609–621.
Mogasale, V.V., et al., 2021. A descriptive analysis of antimicrobial resistance patterns of WHO priority pathogens isolated in children from a tertiary care hospital in India. Sci. Rep. 11, 1–7.
Mohamed, M.M., et al., 2017. Antibacterial effect of gold nanoparticles against Corynebacterium pseudotuberculosis. International journal of veterinary science and medicine 5, 23–29.
Munger, M.A., et al., 2014. In vivo human time-exposure study of orally dosed commercial silver nanoparticles. Nanomed. Nanotechnol. Biol. Med. 10, 1–9.
Najafi, A., 2016. There Is No Escape from the ESKAPE Pathogens.
Nallathamby, P.D., et al., 2010. Study of the multidrug membrane transporter of single living Pseudomonas aeruginosa cells using size-dependent plasmonic nanoparticle optical probes. Biochemistry 49, 5942–5953.
Nikaido, H., 1994. Prevention of drug access to bacterial targets: permeability barriers and active efflux. Science 264, 382–388.
Nikaido, H., Pag`es, J.-M., 2012. Broad-specificity efflux pumps and their role in multidrug resistance of Gram-negative bacteria. FEMS Microbiol. Rev. 36, 340–363.
Nishino, K., et al., 2006. Virulence and drug resistance roles of multidrug efflux systems of Salmonella enterica serovar Typhimurium. Mol. Microbiol. 59, 126–141.
Nishino, K., et al., 2007. Regulation of multidrug efflux systems involved in multidrug and metal resistance of Salmonella enterica serovar Typhimurium. J. Bacteriol. 189, 9066–9075.
Nishino, K., Yamaguchi, A., 2001. Analysis of a complete library of putative drug transporter genes in Escherichia coli. J. Bacteriol. 183, 5803–5812.
Novick, R., 2008. Geisinger e. Quorum sensing in staphylococci. Annu. Rev. Genet. 42, 541–564.
Ohki, R., Tateno, K., 2004. Increased stability of bmr3 mRNA results in a multidrug- resistant phenotype in Bacillus subtilis. J. Bacteriol. 186, 7450–7455.
Okkeh, M., et al., 2021. Gold nanoparticles: can they Be the next magic bullet for multidrug-resistant bacteria? Nanomaterials 11, 312.
Olliver, A., et al., 2005. Overexpression of the multidrug efflux operon acrEF by insertional activation with IS1 or IS10 elements in Salmonella enterica serovar typhimurium DT204 acrB mutants selected with fluoroquinolones. Antimicrob. Agents Chemotherapy 49, 289–301.
Organization, W.H., 2017. Prioritization of Pathogens to Guide Discovery, Research and Development of New Antibiotics for Drug-Resistant Bacterial Infections, Including Tuberculosis. World Health Organization.
Owens Jr., R.C., et al., 2008. Antimicrobial-associated risk factors for Clostridium difficile infection. Clin. Infect. Dis. 46, S19–S31.
Padwal, P., et al., 2014. Polyacrylic acid-coated iron oxide nanoparticles for targeting drug resistance in mycobacteria. Langmuir 30, 15266–15276.
Padwal, P., et al., 2015. Biocompatible citric acid-coated iron oxide nanoparticles to enhance the activity of first-line anti-TB drugs in Mycobacterium smegmatis.
J. Chem. Technol. Biotechnol. 90, 1773–1781.
Paulsen, I.T., et al., 1996. Proton-dependent multidrug efflux systems. Microbiol. Rev.
60, 575–608.
Pelgrift, R.Y., Friedman, A.J., 2013. Nanotechnology as a therapeutic tool to combat microbial resistance. Adv. Drug Deliv. Rev. 65, 1803–1815.
Peters, B.M., et al., 2010. Antimicrobial peptides: primeval molecules or future drugs?
PLoS Pathog. 6 e1001067.
Piddock, L.J., et al., 2010. Natural and synthetic compounds such as trimethoprim behave as inhibitors of efflux in Gram-negative bacteria. J. Antimicrob. Chemother. 65, 1215–1223.
Piddock, L.J., et al., 2000. Evidence for an efflux pump mediating multiple antibiotic resistance in Salmonella enterica serovar Typhimurium. Antimicrob. Agents Chemother. 44, 3118–3121.
Piras, A.M., et al., 2015. Chitosan nanoparticles loaded with the antimicrobial peptide temporin B exert a long-term antibacterial activity in vitro against clinical isolates of Staphylococcus epidermidis. Front. Microbiol. 6, 372.
Poole, K., 2005. Antimicrob chemother. Efflux-mediated antimicrobial resistance 56, 20–51.
Porras Go´mez, M., et al., 2012. Overview of Multidrug-Resistant Pseudomonas aeruginosa and Novel Therapeutic Approaches.
Prabhu, S., Poulose, E., 2012a. Silver nanoparticles: mechanism of antimicrobial action, synthesis, medical applications, and toxicity effects. Int. Nano Lett. 2, 1–10.
Prabhu, S., Poulose, E.K., 2012b. Silver nanoparticles: mechanism of antimicrobial action, synthesis, medical applications, and toxicity effects. Int. Nano Lett. 2, 1–10.
Putman, M., et al., 2000. Molecular properties of bacterial multidrug transporters.
Microbiol. Mol. Biol. Rev. 64, 672–693.
Raafat, D., et al., 2008. Insights into the mode of action of chitosan as an antibacterial compound. Appl. Environ. Microbiol. 74, 3764–3773.
Ramaswamy, V., et al., 2007. Listeria-review of epidemiology and pathogenesis.
J. Microbiol. Immunol. Infect. 40, 4.
Ramirez, M.S., et al., 2013. Rise and dissemination of aminoglycoside resistance: the aac (6')-Ib paradigm. Front. Microbiol. 4, 121.
Randall, L.P., et al., 2001. Association between cyclohexane resistance in Salmonella of different serovars and increased resistance to multiple antibiotics, disinfectants and dyes. J. Med. Microbiol. 50, 919–924.
Rezaei-Zarchi, S., et al., 2012. Study of bactericidal properties of carbohydrate-stabilized platinum oxide nanoparticles. Int. Nano Lett. 2, 1–5.
Ricci, V., et al., 2006. Ciprofloxacin-resistant Salmonella enterica serovar Typhimurium strains are difficult to select in the absence of AcrB and TolC. Antimicrob. Agents Chemother. 50, 38–42.
Roca, I., et al., 2009. CraA, a major facilitator superfamily efflux pump associated with chloramphenicol resistance in Acinetobacter baumannii. Antimicrob.Agents Chemotherapy 53, 4013–4014.
Rosi, N.L., et al., 2006. Oligonucleotide-modified gold nanoparticles for intracellular gene regulation. Science 312, 1027–1030.
Rudramurthy, G.R., et al., 2016. Nanoparticles: alternatives against drug-resistant pathogenic microbes. Molecules 21, 836.
Ruparelia, J.P., et al., 2008. Strain specificity in antimicrobial activity of silver and copper nanoparticles. Acta Biomater. 4, 707–716.
Saha, B., et al., 2007. In vitro structural and functional evaluation of gold nanoparticles conjugated antibiotics. Nanoscale Research Letters 2, 614–622.
Sardari, S., Dezfulian, M., 2007. Cheminformatics in anti-infective agents discovery. Mini Rev. Med. Chem. 7, 181–189.
Scandorieiro, S., et al., 2016. Synergistic and additive effect of oregano essential oil and biological silver nanoparticles against multidrug-resistant bacterial strains. Front. Microbiol. 7, 760.
Schairer, D.O., et al., 2012. The potential of nitric oxide releasing therapies as antimicrobial agents. Virulence 3, 271–279.
Schindler, B.D., Kaatz, G.W., 2016. Multidrug efflux pumps of Gram-positive bacteria.
Drug Resist. Updates 27, 1–13.
Schmitt, L., Tamp´e, R., 2002. Structure and mechanism of ABC transporters. Curr. Opin.
Struct. Biol. 12, 754–760.
Schneiders, T., et al., 2003. Role of AcrR and RamA in fluoroquinolone resistance in clinical Klebsiella pneumoniae isolates from Singapore. Antimicrob.Agents Chemotherapy 47, 2831–2837.
Shah, D., et al., 2006. Persisters: a distinct physiological state of E. coli. BMC Microbiol.
6, 1–9.
Shahverdi, A.R., et al., 2007. Synthesis and effect of silver nanoparticles on the antibacterial activity of different antibiotics against Staphylococcus aureus and Escherichia coli. Nanomed. Nanotechnol. Biol. Med. 3, 168–171.
Sies, H., 1997. Oxidative stress: oxidants and antioxidants. Exp. Physiol.: Translation and Integration 82, 291–295.
Sintubin, L., et al., 2012. Biologically produced nanosilver: current state and future perspectives. Biotechnol. Bioeng. 109, 2422–2436.
Slavin, Y.N., et al., 2021. Novel lignin-capped silver nanoparticles against multidrug- resistant bacteria. ACS Appl. Mater. Interfaces 13, 22098–22109.
Sorres, J., et al., 2018. Ilicicolinic acids and ilicicolinal derivatives from the fungus Neonectria discophora SNB-CN63 isolated from the nest of the termite Nasutitermes corniger found in French Guiana show antimicrobial activity. Phytochemistry 151, 69–77.
Soumet, C., et al., 2005. Screening of benzalkonium chloride resistance in Listeria monocytogenes strains isolated during cold smoked fish production. Lett. Appl. Microbiol. 41, 291–296.
Spengler, G., et al., 2017. New roads leading to old destinations: efflux pumps as targets to reverse multidrug resistance in bacteria. Molecules 22, 468.
Srinivasan, V.B., et al., 2014. Role of novel multidrug efflux pump involved in drug resistance in Klebsiella pneumoniae. PloS One 9 e96288.
Srinivasan, V.B., et al., 2006. Mechanism of drug resistance in clonally related clinical isolates of Vibrio fluvialis isolated in Kolkata, India. Antimicrob.Agents Chemotherapy 50, 2428–2432.
Stavri, M., et al., 2007. Bacterial efflux pump inhibitors from natural sources.
J. antimicrobial chemotherapy 59, 1247–1260.
Stock, I., et al., 2003. Natural antibiotic susceptibility of strains of Serratia marcescens and the S. liquefaciens complex: S. liquefaciens sensu stricto, S. proteamaculans and
S. grimesii. Int. J. Antimicrob. Agents 22, 35–47.
Strateva, T., et al., 2007. Problematic clinical isolates of Pseudomonas aeruginosa from the university hospitals in Sofia, Bulgaria: current status of antimicrobial resistance and prevailing resistance mechanisms. J. Med. Microbiol. 56, 956–963.
Su, X.-Z., et al., 2005. AbeM, an H -coupled Acinetobacter baumannii multidrug efflux pump belonging to the MATE family of transporters. Antimicrob.Agents Chemotherapy 49, 4362–4364.
Sugawara, E., et al., 2006. Pseudomonas aeruginosa porin OprF exists in two different conformations. J. Biol. Chem. 281, 16220–16229.
Sun, J., et al., 2014. Biochemical and Biophysical Research Communications Bacterial multidrug efflux pumps: mechanisms, physiology and pharmacological exploitations. Biochem. Biophys. Res. Commun. 453, 254–267.
Takatsuka, Y., et al., 2010. Mechanism of recognition of compounds of diverse structures by the multidrug efflux pump AcrB of Escherichia coli. Proc. Natl. Acad. Sci. Unit. States Am. 107, 6559–6565.
Takatsuka, Y., Nikaido, H., 2009. Covalently linked trimer of the AcrB multidrug efflux pump provides support for the functional rotating mechanism. J. Bacteriol. 191, 1729–1737.
Tanagawa, M., et al., 1999. Inhibitory effect of antibacterial resin composite against Streptococcus mutans. Caries Res. 33, 366–371.
Tin, S., et al., 2009. Activity of Chitosans in combination with antibiotics in Pseudomonas aeruginosa. Int. J. Biol. Sci. 5, 153.
Tran, N., et al., 2010. Bactericidal effect of iron oxide nanoparticles on Staphylococcus aureus. Int. J. Nanomed. 5, 277.
Truong-Bolduc, Q.C., Hooper, D.C., 2007. The transcriptional regulators NorG and MgrA modulate resistance to both quinolones and ß-lactams in Staphylococcus aureus.
J. Bacteriol. 189, 2996–3005.
Upadya, M., et al., 2011. Role of efflux pump inhibitors on the antibiofilm efficacy of calcium hydroxide, chitosan nanoparticles, and light-activated disinfection.
J. Endod. 37, 1422–1426.
Usman, M.S., et al., 2013. Synthesis, characterization, and antimicrobial properties of copper nanoparticles. Int. J. Nanomed. 8, 4467.
Venubabu Thati, A., et al., 2010. Nanostructured zinc oxide enhances the activity of antibiotics against Staphylococcus aureus. J. Biosci Tech. 1, 64–69.
Waters, C.M., Bassler, B.L., 2005. Quorum sensing: cell-to-cell communication in bacteria. Annu. Rev. Cell Dev. Biol. 21, 319–346.
Wijnhoven, S.W., et al., 2009. Nano-silver–a review of available data and knowledge gaps in human and environmental risk assessment. Nanotoxicology 3, 109–138.
Wright, G.D., 2011. Molecular mechanisms of antibiotic resistance. Chem. Commun. 47, 4055–4061.
Wright, G.D., 2016. Antibiotic adjuvants: rescuing antibiotics from resistance. Trends Microbiol. 24, 862–871.
Xing, R., et al., 2013. Hollow iron oxide nanoparticles as multidrug resistant drug delivery and imaging vehicles. Nano research 6, 1–9.
Xu, G.-M., 2016. Relationships between the regulatory systems of quorum sensing and multidrug resistance. Front. Microbiol. 7, 958.
Xu, X.-H.N., et al., 2004. Real-time probing of membrane transport in living microbial cells using single nanoparticle optics and living cell imaging. Biochemistry 43, 10400–10413.
Yamanaka, M., et al., 2005. Bactericidal actions of a silver ion solution on Escherichia coli, studied by energy-filtering transmission electron microscopy and proteomic analysis. Appl. Environ. Microbiol. 71, 7589–7593.
Yang, X., et al., 2021. Exploitation of antimicrobial nanoparticles and their applications in biomedical engineering. Appl. Sci. 11, 4520.
Yoshida, K., et al., 1999. Characterization and inhibitory effect of antibacterial dental resin composites incorporating silver-supported materials. J. Biomed. Mater. Res.: An Official Journal of The Society for Biomaterials 47, 516–522. The Japanese
"Nobody ever went broke underestimating the intelligence of the
American public."
H. L. Mencken
"It’s easier to fool people than to convince them that they have been fooled."
Anon
Recent IPIX News
- Form 8-K - Current report • Edgar (US Regulatory) • 02/01/2024 01:30:25 PM
- Form 8-K - Current report • Edgar (US Regulatory) • 12/05/2023 09:25:58 PM
- Form 10-Q - Quarterly report [Sections 13 or 15(d)] • Edgar (US Regulatory) • 11/20/2023 09:05:44 PM
- Form NT 10-Q - Notification of inability to timely file Form 10-Q or 10-QSB • Edgar (US Regulatory) • 11/15/2023 01:00:19 PM
- Form 8-K - Current report • Edgar (US Regulatory) • 10/30/2023 08:15:25 PM
- Form 10-K - Annual report [Section 13 and 15(d), not S-K Item 405] • Edgar (US Regulatory) • 09/28/2023 01:00:08 PM
VHAI - Vocodia Partners with Leading Political Super PACs to Revolutionize Fundraising Efforts • VHAI • Sep 19, 2024 11:48 AM
Dear Cashmere Group Holding Co. AKA Swifty Global Signs Binding Letter of Intent to be Acquired by Signing Day Sports • DRCR • Sep 19, 2024 10:26 AM
HealthLynked Launches Virtual Urgent Care Through Partnership with Lyric Health. • HLYK • Sep 19, 2024 8:00 AM
Element79 Gold Corp. Appoints Kevin Arias as Advisor to the Board of Directors, Strengthening Strategic Leadership • ELMGF • Sep 18, 2024 10:29 AM
Mawson Finland Limited Further Expands the Known Mineralized Zones at Rajapalot: Palokas step-out drills 7 metres @ 9.1 g/t gold & 706 ppm cobalt • MFL • Sep 17, 2024 9:02 AM
PickleJar Announces Integration With OptCulture to Deliver Holistic Fan Experiences at Venue Point of Sale • PKLE • Sep 17, 2024 8:00 AM