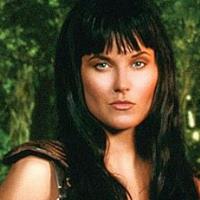
Tuesday, July 14, 2020 8:24:38 AM
Crosstalk Between Hypoxia and ER Stress Response: A Key Regulator of Macrophage Polarization
Paula Díaz-Bulnes1†, María Laura Saiz1†, Carlos López-Larrea1,2* and Ramón M. Rodríguez1
1Translational Immunology Laboratory, Health Research Institute of the Principality of Asturias, Hospital Universitario Central de Asturias, Oviedo, Spain
2Immunology Service, Hospital Universitario Central de Asturias, Oviedo, Spain
Macrophage activation and polarization are closely linked with metabolic rewiring, which is required to sustain their biological functions. These metabolic alterations allow the macrophages to adapt to the microenvironment changes associated with inflammation or tissue damage (hypoxia, nutrient imbalance, oxidative stress, etc.) and to fulfill their highly energy-demanding proinflammatory and anti-microbial functions. This response is integrated via metabolic sensors that coordinate these metabolic fluxes with their functional requirements. Here we review how the metabolic and phenotypic plasticity of macrophages are intrinsically connected with the hypoxia stress sensors and the unfolded protein response in the endoplasmic reticulum, and how these molecular pathways participate in the maladaptive polarization of macrophages in human pathology and chronic inflammation.
Introduction
Macrophages are highly plastic immune cells involved in tissue homeostasis, immune response and inflammation. In recent years, numerous studies have demonstrated that macrophage plasticity is closely linked to a metabolic adaptation that supports the energy requirements and cellular growth associated with these functions (1). In the inflammatory milieu, stimulated macrophages face challenging metabolic conditions in which they must compete for nutrients and oxygen with other immune cells or pathogens invading the tissue. In this environment, macrophages must generate sufficient energy output to sustain growth, phagocytosis, antimicrobial functions, and also to fuel the highly demanding synthesis of inflammatory mediators that contribute to the propagation or resolution of the inflammatory response. In a simplified view, macrophage polarization states have been defined as proinflammatory M1 (classically activated) or anti-inflammatory M2 (alternatively activated), a classification that mirrors the Th1/Th2 polarization of T cells (2). The definition of these activation states contributed to the understanding and categorization of the opposing functions of macrophages: killing and repairing (3). However, in reality, the dichotomous M1/M2 distinction is not representative of what takes place in an in vivo setting, as M1 and M2 stimuli do not exist alone in tissues. Instead, the macrophage population represents a continuum of phenotypes that stands between these two extremes, implying that discrete populations should not be so crudely depicted (4). Indeed, transcriptome analysis of stimulated macrophages with different activation signals show that these cells experience a transcriptional reprogramming that extends the M1/M2 paradigm (5). However, since most of the preceding literature has used the nomenclature based on the M1/M2 classification as a tool for dissecting the complex macrophage phenotypes, this terminology has been maintained throughout some parts of this review.
Pathological situations in which nutrient availability is compromised, such as infection, chronic inflammation, diseases associated with metabolic/nutrient imbalance (diabetes, obesity, atherosclerosis) or ischemia/reperfusion events associated with organ transplantation or surgery, generate metabolic stress that potentially subverts macrophage functions to induce maladaptive polarization states (6–8). Macrophages can perceive these signals in the tissue microenvironment via metabolic sensors that coordinate metabolic and transcriptomic rewiring and are therefore very responsive to any abnormal imbalance associated with pathology. For instance, hypoxic (oxygen-limiting) environments associated with inflammation or ischemia activate cellular sensors for oxygen and the hypoxia-inducible factor (HIF), which induce a metabolic switch from oxidative to glycolytic metabolism and proinflammatory polarization that further exacerbates the inflammatory response (9, 10). This hypoxic environment is also closely linked to an endoplasmic reticulum (ER) stress response, which is critical for the integration of the metabolic and inflammatory responses in macrophages. The ER organelle plays a central role in cellular nutrient sensing, activating the signaling pathway called the unfolded protein response (UPR) under metabolic stress conditions such as hypoxia or nutrient imbalance (amino acid or glucose deprivation, infectious process, etc.). This response is partially mediated by the mTORC1 pathway, which is a positive regulator of protein synthesis, and cell growth that coordinates the cellular balance between anabolic pathways and energy consumption in macrophages (11).
Considering all this evidence, it is clear that cellular sensors for oxygen and ER stress pathways contribute critically to the signal integration and metabolic adaptation associated with various pathological conditions. In this context, macrophage polarization lies at the intersection between metabolic imbalance and inflammation, and understanding the molecular pathways connecting these processes will be critical for the development of new therapeutic strategies. Here, we review how ER stress and hypoxic responses are organized and connected with macrophage function, focusing particularly on the maladaptive polarization states associated with the pathological contexts in which the metabolic balance in macrophages is compromised.
Molecular Mechanisms in ER Stress: Unfolded Protein Response
The ER has a crucial role in maintaining cellular functions, such as protein folding, maturation and assembly of proteins that are trafficked along the secretory pathway, as well as preserving cellular calcium homeostasis. Several physiological and pathological conditions involving imbalance in ER folding capacity, accumulation of misfolded proteins, hypoxia, amino acid or glucose deprivation, oxidative stress, viral infection or disruption of ER calcium balance can trigger ER stress and activate the UPR that maintains cellular homeostasis and cell survival (12). This mechanism rescues the cells from the damage caused by ER stress, and in the event of unresolvable stress, induces apoptosis. The UPR comprises three major signaling pathways, which are initiated by the activation of three protein sensors: activating transcription factor 6 (ATF6), pancreatic eukaryotic translation initiation factor 2a (eIF2a) kinase (PERK), and inositol-requiring enzyme 1a (IRE1a). Under normal conditions, these sensors are bound to glucose-regulated protein 78 (GRP78), an ER chaperone, also known as BiP (binding immunoglobulin protein), that maintains them in an inactive state. Under ER stress conditions, GRP78 dissociates from the sensors and binds to unfolded proteins (13), allowing activation by dimerization or translocation (Figure 1). Accordingly, activated IRE1a performs two enzymatic functions upon dimerization: serine/threonine kinase and endoribonuclease (RNase) activity (14). This RNase domain in IRE1a initiates the non-conventional splicing of XBP1, which produces a translational frameshift and creates XBP1s (spliced), a potent transcriptional activator (15). XBP1s is translocated to the nucleus and induces the transcription of an extensive variety of chaperones and enzymes that together increase ER size and function. During prolonged ER stress, the beneficial effects of IRE1a activation through the activity of XBP1s may be reduced and could activate the properties of IRE1a to promote inflammation and cell death (16). Similar to IRE1a, dissociation of GRP78 from the ER luminal domain of PERK induces activation through homodimerization and autophosphorylation. Activated PERK phosphorylates the eukaryotic translation initiation factor (eIF2a), which reduces the degree of recognition and translation of the AUG initiation codon, resulting in a lower protein load on the damaged ER (17). Remarkably, eIF2a can be phosphorylated independently of ER stress by the general control non-derepressible 2 (GCN2) kinase, which is induced by amino acid deprivation (18). This results in the activation of ATF4, Nrf2 (nuclear erythroid 2 p45-related factor 2), and NF-?B (nuclear factor kappa ß), a master transcription factor with numerous functions, including regulation of the inflammatory response (19). ATF4 also activates the transcription of C/EBP homologous protein (CHOP), which is involved in ER-stress-mediated apoptosis and cell death (20). The third arm of the pathway relies on ATF6, a type II transmembrane protein. There are two isoforms of ATF6 (ATF6a and ATF6ß), but only ATF6a is required to activate UPR. When ATF6 senses ER stress, it is transported to the Golgi, where it is cleaved by site 1 and site 2 proteases (S1P and S2P) (21). The cleaved soluble cytosolic fragment can now enter the nucleus and trigger the expression of genes encoding proteins such as GRP78, GRP94, p58IPK, and XBP1 that function to increase ER capacity and folding as well as the expression of genes of the ERAD pathway involved in protein degradation (22, 23). Of note, cleaved ATF6 can act as an enhancer to increase the CREBH-mediated (cAMP response element-binding protein H) acute inflammatory response (24), representing a link between ATF6 and inflammation.
Continued...
https://www.frontiersin.org/articles/10.3389/fimmu.2019.02951/full
Recent AVXL News
- ZenaTech, Inc. (NASDAQ: ZENA) To Commence Trading Today • InvestorsHub NewsWire • 10/01/2024 11:00:00 AM
- Anavex Life Sciences to Present at the H.C. Wainwright 26th Annual Global Investment Conference 2024 • GlobeNewswire Inc. • 09/03/2024 11:30:00 AM
- Form 10-Q - Quarterly report [Sections 13 or 15(d)] • Edgar (US Regulatory) • 08/06/2024 09:21:05 PM
- Anavex Life Sciences Reports Fiscal 2024 Third Quarter Financial Results and Provides Business Update • GlobeNewswire Inc. • 08/06/2024 11:30:00 AM
- Anavex Life Sciences to Announce Fiscal 2024 Third Quarter Financial Results on Tuesday, August 6, 2024 • GlobeNewswire Inc. • 08/01/2024 11:30:00 AM
- Form 8-K - Current report • Edgar (US Regulatory) • 07/30/2024 09:20:53 PM
- Anavex Life Sciences Announces Translational Biomarker Data for ANAVEX®2-73 (blarcamesine) in Fragile X Syndrome (Major Cause of Autism) at the 19th NFXF International Fragile X Conference • GlobeNewswire Inc. • 07/30/2024 11:30:00 AM
- Form S-3 - Registration statement under Securities Act of 1933 • Edgar (US Regulatory) • 07/29/2024 09:21:49 PM
- Results from Anavex Life Sciences Landmark Phase IIb/III Trial of Blarcamesine Presented at Alzheimer's Association Conference • GlobeNewswire Inc. • 07/28/2024 09:00:00 PM
- Anavex Life Sciences to Present at the H.C. Wainwright 5th Annual Neuro Perspectives Virtual Conference • GlobeNewswire Inc. • 06/20/2024 11:30:00 AM
- Form 4 - Statement of changes in beneficial ownership of securities • Edgar (US Regulatory) • 06/17/2024 11:30:10 AM
- Anavex Life Sciences Announces Expansion of Leadership Team • GlobeNewswire Inc. • 05/22/2024 11:30:00 AM
- Form 4 - Statement of changes in beneficial ownership of securities • Edgar (US Regulatory) • 05/17/2024 10:01:00 AM
- Anavex Life Sciences to Present at the H.C. Wainwright 2nd BioConnect Investor Conference at NASDAQ • GlobeNewswire Inc. • 05/14/2024 11:30:00 AM
- Shareholders that lost money on Anavex Life Sciences Corporation(AVXL) Urged to Join Class Action - Contact The Gross Law Firm to Learn More • PR Newswire (US) • 05/10/2024 09:45:00 AM
- Form 10-Q - Quarterly report [Sections 13 or 15(d)] • Edgar (US Regulatory) • 05/09/2024 08:35:55 PM
- Form 8-K - Current report • Edgar (US Regulatory) • 05/09/2024 12:00:30 PM
- Anavex Life Sciences Reports Fiscal 2024 Second Quarter Financial Results and Provides Business Update • GlobeNewswire Inc. • 05/09/2024 11:30:00 AM
- The Gross Law Firm Announces the Filing of a Securities Class Action on Behalf of Anavex Life Sciences Corporation(AVXL) Shareholders • PR Newswire (US) • 05/07/2024 09:45:00 AM
- Form DEFA14A - Additional definitive proxy soliciting materials and Rule 14(a)(12) material • Edgar (US Regulatory) • 05/06/2024 10:03:33 AM
- Anavex Life Sciences Corporation Sued for Securities Law Violations - Contact The Gross Law Firm Before May 13, 2024 to Discuss Your Rights - AVXL • PR Newswire (US) • 05/03/2024 09:45:00 AM
- Anavex Life Sciences to Announce Fiscal 2024 Second Quarter Financial Results on Thursday, May 9th, 2024 • GlobeNewswire Inc. • 05/02/2024 11:30:00 AM
- May 13, 2024 Deadline: Contact The Gross Law Firm to Join Class Action Suit Against AVXL • PR Newswire (US) • 04/26/2024 09:45:00 AM
- Contact The Gross Law Firm by May 13, 2024 Deadline to Join Class Action Against Anavex Life Sciences Corporation(AVXL) • PR Newswire (US) • 04/19/2024 09:45:00 AM
North Bay Resources Announces Assays up to 5 oz/ton Gold, 1.5 oz/ton Platinum, 0.5 oz/ton Palladium, and 0.5 oz/ton Rhodium at Mt. Vernon Gold Mine, Sierra County, California • NBRI • Oct 4, 2024 9:15 AM
Basanite, Inc. Appoints Ali Manav as Interim Chief Executive Officer • BASA • Oct 3, 2024 9:15 AM
Integrated Ventures Announces Launch of MedWell Facilities, LLC and Lease Agreement with Giant Fitness Clubs • INTV • Oct 3, 2024 8:45 AM
Beyond the Horizon: Innovative Drug Combinations Offer New Hope for Alzheimer's and More • NVS • Oct 3, 2024 8:45 AM
SMX and FinGo Enter Into Collaboration Mandate to Develop a Joint 'Physical to Digital' Platform Service To Enhance Natural Rubber Industry's Ability to Report on Sustainable and Ethical Supply Chains • SMX • Oct 3, 2024 7:00 AM
Transforming Alzheimer's Treatment: Innovative Combinations to Boost Cognition • PFE • Oct 2, 2024 9:00 AM