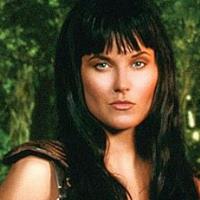
Tuesday, March 10, 2020 12:48:27 PM
If there are biomarkers, improved sleep being the most obvious, that prove that GABA/glutamate balance is improving all three will be approved provisionally based on the Rett's data and EHR evidence from all three indications. This would be why they needed an arm of all three trials in Australia. Positive GABA/glutamate data from the upcoming Rett's trial is extremely likely given the response of the older girls in this trial, we've known this since September:
https://www.anavex.com/anavex-life-sciences-announces-preliminary-clinical-efficacy-data-of-its-u-s-phase-2-clinical-trial-of-anavex2-73-in-patients-with-rett-syndrome/
This could easily have been the trigger for 4th quarter institutional buying.
GABA/glutamate balance (homeostasis) is key in every CNS disorder that I can think of.
1. Introduction
Glutamate and gamma-aminobutyric acid (GABA) are the major neurotransmitters in the brain. Inhibitory GABA and excitatory glutamate work together to control many processes, including the brain’s overall level of excitation. A balanced interaction is required to maintain the physiological homeostasis, while prolonged imbalance can lead to disease. Glutamatergic/GABAergic imbalance can be found in autism spectrum disorders and anxiety disorders with elevated glutamatergic neurotransmission, while high levels of GABA produce more relaxation and even sedation. Neurotransmitter levels can be affected by external factors, for example, alcohol. Alcohol potentiates the sedentary effects of GABA, while inhibiting the excitatory aspects of glutamate, resulting in an overall increase in GABA/glutamate ratio. This leads to sensations of relaxation and in later stages to loss of control with slurred speech, unsteady gait and loss of social anxiety. The GABA/glutamate balance can also be affected by autoimmunity and genetic disorders. The contributions of GABA and glutamate in extra-neuronal signaling are by far less recognized. We will discuss extra-neuronal GABAergic and glutamatergic signaling and its relevance in insulin secretion from the pancreatic islets of Langerhans.
2. Glutamate and GABA during development
Both glutamatergic and GABAergic neurons are highly diversified in the central nervous system (CNS). More than half a century after the discovery of the effects of GABA, it is now established that in mature neurons, neuronal excitability is characterized by a balance between glutamatergic excitatory input and GABAergic inhibitory transmission. This balance is reached during development. However, the functions of GABAergic signaling are not restricted to a pure inhibitory mechanism at the synaptic level. This is a too simplistic view. For instance, GABA influences patterns and oscillations that are very relevant from the behavioral point of view [1]. GABA and glutamate expression are already widespread in the embryonic stage, and glutamate receptors are expressed in neurons even before glutamatergic synaptogenesis [1]. While glutamate receptor activities tune the developing GABAergic synapse [2], GABA is now considered the main excitatory transmitter during early development, acting not only at a synaptic and network level, but also on cell cycle and migration [1]. This excitatory function of GABA is caused by elevated neuronal intracellular chloride concentration at the early stages of development. The efflux of chloride mediated by GABA in immature neurons is excitatory, triggering sodium spikes and activating voltage-gated Ca2+ channels [1]. With time, a progressive reduction of chloride efflux occurs. This explains the shift from a depolarizing to a hyperpolarizing effect. Obata and colleagues were the first to suggest this developmentally regulated shift at the level of the spinal cord [3]. Neuronal chloride homeostasis is regulated by channels, exchangers and co-transporters. The developmental changes of sodium-potassium-chloride cotransporter 1 (NKCC1) (ensuring chloride uptake; higher expression in immature neurons) and potassium-chloride transporter 2 (KCC2) (principal chloride extruder; higher expression in mature neurons) are the masterpieces for the changes in chloride efflux associated with maturation. The developmental shift from local to large-scale network activity occurs in parallel with a gradual shift from electrical to chemical synaptic transmission [4].
It is noteworthy that in immature neurons activation of GABAA receptors leads to an increase in the intra-cellular concentration of Ca2+, as a consequence of the stimulation of voltage-gated Ca2+ channels, which exerts trophic effects on neuritic growth, migration and synaptogenesis. Blocking the GABAA receptor reduces the cytoplasmic concentrations of Ca2+ [5]. In addition, the activation of GABAB receptors depresses the GABAA receptor-mediated Ca2+ increase and therefore the GABAB pathway is likely supervising the entry of Ca2+ [6]. In granule cells of the cerebellum, the changes in the concentrations of Ca2+ outlast the exposure to GABA by several minutes [7]. The GABAA-activated Ca2+ influx regulates the expression of the chloride extruder KCC2 [8]. One example of the relevance of this physiological shift in the chloride gradient occurs during delivery when the maternal hormone oxytocin triggers labor [9].
The electrical activity of neurons is a guide for the genesis of neuronal connections. Indeed, neuronal activity exerts a key role in the development of inhibitory GABAergic synapses, interacting closely with genetic programs. Blocking neuronal activity in developing neurons decreases the density of inhibitory synapses, confirming an activity-dependent development [10, 11]. The expression of GABAergic plasticity is related to modifications in the quantity of neurotransmitter in individual vesicles. Migrating neurons express, already at an early stage, both GABA and glutamate receptors [1], but GABA receptors are likely to be established first [12]. Interestingly, tangentially migrating interneurons express AMPA but not GABA or NMDA receptors. Therefore, a modulation/targeting of neurons via selective activation of receptors can be achieved [13]. This has implications for understanding and treatment of migrating disorders affecting the nervous system.
The building of brain networks is a highly complex task which requires organized sequential events, both spatially and from a timing standpoint. While an overdrive of GABAergic signaling slows the development, the overactivity of glutamatergic signaling causes excitotoxicity [1]. GABA receptors are the first to be active, even when synapses are still non-operant. This creates a shunting effect preventing excitotoxicity, since the Na+ and Ca2+ spikes triggered by GABA require only a 30–40 mV driving force. Such a shunt is part of the synergistic interactions between GABA and glutamate. Thanks to these interactions, the neuronal networks in development generate primitive patterns of discharges, observed in vivo and in cultured networks, such as the giant depolarizing potentials (GDPs) which allow the building of functional units [1]. GDPs resemble interictal-like epileptiform discharges and provide synchronous Ca2+ oscillations also contributing to the development of networks. GDPs rely on the release of GABA, glutamate, and glycine at the onset of synaptogenesis [14]. The synchronized activity is one of the factors controlling the phenomenon of maturation [11]. Synchronization is also achieved, thanks to gap junctions, intrinsic voltage-dependent conductance [15], and non-vesicular paracrine release of neurotransmitters [16].
The capacity of developing nervous system to generate spontaneous activity in absence of external stimulation is a remarkable feature that has been observed in particular in the retina, the cerebral cortex, the hippocampus, the cerebellum, the hindbrain, and the spinal cord [14]. Recent works highlight that network bursts are driven by AMPA pathways in terms of coordination, whereas the shaping of the dynamics of spiking activities is regulated by NMDA- and GABA-associated currents [17].
3. Glutamate and GABA in the mature mammalian brain
3.1. Biosynthesis of glutamate and GABA: the glutamate/GABA-glutamine cycle
Glutamate and GABA do not cross the blood-brain barrier and must therefore be synthesized within the CNS. As neurons lack the enzyme pyruvate carboxylase and therefore cannot synthesize glutamate through the TCA cycle [18], they rely on astrocytes for the generation of glutamate. Astrocytes generate glutamate via de novo synthesis or by “recycling” glutamine from GABA and glutamate after reuptake. However, de novo synthesis makes up only ~15% of astrocytic glutamate [19]. In this reaction, pyruvate is generated from glucose during glycolysis and enters the TCA after conversion to Acetyl CoA. The TCA product a-ketoglutarate can be converted to glutamate, which is converted to glutamine by glutamine synthetase, an enzyme that is predominately, if not exclusively, located in astrocytes [20]. Glutamine exits astrocytes via the bidirectional N system transporters, SNAT3 and SNAT5 [21], and enter neurons via the unidirectional system A transporters, SNAT1, SNAT2 [21], and SNAT7 [22]. There glutamine is converted back to glutamate by phosphate-activated glutaminase, an enzyme which is expressed preferentially in neurons [23]. GABAergic neurons require an additional step to convert glutamate to GABA through decarboxylation. After release from the neurons, GABA and glutamate reenter the astrocytes to be “recycled” to glutamine. A small portion of glutamate is oxidatively metabolized, thus making de novo synthesis of glutamate necessary to maintain adequate glutamate levels [24]. The continuous recycling of glutamate, GABA and glutamine between neurons and astrocytes is known as the glutamate/GABA-glutamine cycle [25] (Figure 1).
More...
https://www.intechopen.com/books/gaba-and-glutamate-new-developments-in-neurotransmission-research/gaba-and-glutamate-their-transmitter-role-in-the-cns-and-pancreatic-islets
See also this post:
Biology concepts for Alzheimer’s disease (2/20/2020)
courtesy of amstocks82
https://investorshub.advfn.com/boards/read_msg.aspx?message_id=153974885
Recent AVXL News
- Anavex Life Sciences Announces Submission of Blarcamesine MAA for Treatment of Alzheimer’s Disease to EMA • GlobeNewswire Inc. • 11/26/2024 12:30:00 PM
- Anavex Life Sciences Announces Acceptance of Peer-Reviewed Manuscript of Oral Blarcamesine Phase IIb/III Data in a Reference Alzheimer’s Disease Journal • GlobeNewswire Inc. • 11/25/2024 12:30:00 PM
- Anavex’s Blarcamesine Achieves Pre-specified Efficacy in Phase IIb/III Alzheimer’s Trial: Data Presented at CTAD Conference 2024 • GlobeNewswire Inc. • 10/31/2024 08:00:00 AM
- ZenaTech, Inc. (NASDAQ: ZENA) Software Company Acquisition • InvestorsHub NewsWire • 10/23/2024 12:03:31 PM
- Anavex Life Sciences Announces Encouraging Preliminary Biomarker Results from Ongoing Phase 2 Study of ANAVEX®3-71 for the Treatment of Schizophrenia • GlobeNewswire Inc. • 10/17/2024 11:30:00 AM
- ZenaTech, Inc. (NASDAQ: ZENA) First US Trial of IQ Nano Drone for Inventory Management • InvestorsHub NewsWire • 10/15/2024 12:21:48 PM
- ZenaTech, Inc. (NASDAQ: ZENA) To Commence Trading Today • InvestorsHub NewsWire • 10/01/2024 11:00:00 AM
- Anavex Life Sciences to Present at the H.C. Wainwright 26th Annual Global Investment Conference 2024 • GlobeNewswire Inc. • 09/03/2024 11:30:00 AM
- Form 10-Q - Quarterly report [Sections 13 or 15(d)] • Edgar (US Regulatory) • 08/06/2024 09:21:05 PM
- Anavex Life Sciences Reports Fiscal 2024 Third Quarter Financial Results and Provides Business Update • GlobeNewswire Inc. • 08/06/2024 11:30:00 AM
- Anavex Life Sciences to Announce Fiscal 2024 Third Quarter Financial Results on Tuesday, August 6, 2024 • GlobeNewswire Inc. • 08/01/2024 11:30:00 AM
- Form 8-K - Current report • Edgar (US Regulatory) • 07/30/2024 09:20:53 PM
- Anavex Life Sciences Announces Translational Biomarker Data for ANAVEX®2-73 (blarcamesine) in Fragile X Syndrome (Major Cause of Autism) at the 19th NFXF International Fragile X Conference • GlobeNewswire Inc. • 07/30/2024 11:30:00 AM
- Form S-3 - Registration statement under Securities Act of 1933 • Edgar (US Regulatory) • 07/29/2024 09:21:49 PM
- Results from Anavex Life Sciences Landmark Phase IIb/III Trial of Blarcamesine Presented at Alzheimer's Association Conference • GlobeNewswire Inc. • 07/28/2024 09:00:00 PM
- Anavex Life Sciences to Present at the H.C. Wainwright 5th Annual Neuro Perspectives Virtual Conference • GlobeNewswire Inc. • 06/20/2024 11:30:00 AM
- Form 4 - Statement of changes in beneficial ownership of securities • Edgar (US Regulatory) • 06/17/2024 11:30:10 AM
- Anavex Life Sciences Announces Expansion of Leadership Team • GlobeNewswire Inc. • 05/22/2024 11:30:00 AM
- Form 4 - Statement of changes in beneficial ownership of securities • Edgar (US Regulatory) • 05/17/2024 10:01:00 AM
- Anavex Life Sciences to Present at the H.C. Wainwright 2nd BioConnect Investor Conference at NASDAQ • GlobeNewswire Inc. • 05/14/2024 11:30:00 AM
- Shareholders that lost money on Anavex Life Sciences Corporation(AVXL) Urged to Join Class Action - Contact The Gross Law Firm to Learn More • PR Newswire (US) • 05/10/2024 09:45:00 AM
- Form 10-Q - Quarterly report [Sections 13 or 15(d)] • Edgar (US Regulatory) • 05/09/2024 08:35:55 PM
- Form 8-K - Current report • Edgar (US Regulatory) • 05/09/2024 12:00:30 PM
- Anavex Life Sciences Reports Fiscal 2024 Second Quarter Financial Results and Provides Business Update • GlobeNewswire Inc. • 05/09/2024 11:30:00 AM
Cannabix Technologies Receives Positive Testing Certification for Breath Logix Autonomous Alcohol Breathalyzer • BLO • Nov 26, 2024 8:48 AM
BioQuest, Inc. and BotMakers Expand AI Marketing Services with SpeedLeads XL and MarketScope XL. Bioquest Corp. and BotMakers, Inc. are Partnered to Expand AI Marketing Services with SpeedLead XL and MarketScope XL • BQST • Nov 26, 2024 8:00 AM
BNCM Announces its Q3 and 9-Month Growth Results for 2024 • BNCM • Nov 26, 2024 7:03 AM
FoodTech Trailblazer Beyond Oil Appoints New COO from KFC Amidst Massive Global Expansion (OTCQB: BEOLF) (CSE: BOIL), up 250% this year • BOIL • Nov 25, 2024 11:12 AM
UC Asset announces plan of acquisition to increase awareness for its stock trading • UCASU • Nov 25, 2024 9:30 AM
HealthLynked Receives $500,000 Payment Milestone from Sale of ACO Health Partners • HLYK • Nov 25, 2024 8:00 AM