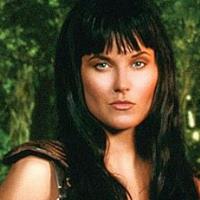
Tuesday, March 19, 2019 12:22:49 PM
Epigenomics. 2013;5(5):583-92. doi: 10.2217/epi.13.54.
Role of epigenetics in Rett syndrome.
Kubota T1, Miyake K, Hirasawa T.
Author information
Abstract
Rett syndrome (RTT) is an X-linked neurodevelopmental disease caused by MECP2 mutations. The MeCP2 protein was originally thought to function as a transcription repressor by binding to methylated CpG dinucleotides, but is now also thought to be a transcription activator. Recent studies suggest that MeCP2 is not only being expressed in neurons, but also in glial cells, which suggests a new paradigm for understanding the pathogenesis of RTT. It has also been demonstrated that reintroduction of MeCP2 into behaviorally affected Mecp2-null mice after birth rescues neurological symptoms, which indicates that epigenetic failures in RTT are reversible. Therefore, RTT may well be seen as a model disease that can be potentially treated by taking advantage of the reversibility of epigenetic phenomena in various congenital neurodevelopmental diseases that were previously thought to be untreatable.
PMID: 24059803 DOI: 10.2217/epi.13.54
https://www.ncbi.nlm.nih.gov/pubmed/24059803
Neural Plasticity
Volume 2012, Article ID 415825, 10 pages
dx.doi.org/10.1155/2012/415825
Review Article
Linking Epigenetics to Human Disease and Rett Syndrome: The Emerging Novel and Challenging Concepts in MeCP2 Research
Robby Mathew Zachariah1,2 and Mojgan Rastegar1,2,3
1Regenerative Medicine Program, University of Manitoba, 745 Bannatyne Avenue, Winnipeg, MB, Canada R3E 0J9
2Department of Biochemistry and Medical Genetics, University of Manitoba, 745 Bannatyne Avenue, Winnipeg, MB, Canada R3E 0J9
3Department of Immunology, University of Manitoba, 745 Bannatyne Avenue, Winnipeg, MB, Canada R3E 0J9
Received 31 August 2011; Accepted 15 November 2011
Academic Editor: Hansen Wang
Copyright © 2012 Robby Mathew Zachariah and Mojgan Rastegar. This is an open access article distributed under the Creative Commons Attribution License, which permits unrestricted use, distribution, and reproduction in any medium, provided the original work is properly cited.
Abstract
Epigenetics refer to inheritable changes beyond DNA sequence that control cell identity and morphology. Epigenetics play key roles in development and cell fate commitments and highly impact the etiology of many human diseases. A well-known link between epigenetics and human disease is the X-linked MECP2 gene, mutations in which lead to the neurological disorder, Rett Syndrome. Despite the fact that MeCP2 was discovered about 20 years ago, our current knowledge about its molecular function is not comprehensive. While MeCP2 was originally found to bind methylated DNA and interact with repressor complexes to inhibit and silence its genomic targets, recent studies have challenged this idea. Indeed, depending on its interacting protein partners and target genes, MeCP2 can act either as an activator or as a repressor. Furthermore, it is becoming evident that although Rett Syndrome is a progressive and postnatal neurological disorder, the consequences of MeCP2 deficiencies initiate much earlier and before birth. To comprehend the novel and challenging concepts in MeCP2 research and to design effective therapeutic strategies for Rett Syndrome, a targeted collaborative effort from scientists in multiple research areas to clinicians is required.
1. Introduction
The term epigenetics refers to inheritable changes in gene expression that control cellular phenotype and fate decisions without alterations in the underlying DNA sequence [1]. In eukaryotes, two main epigenetic regulations are exerted through modifications on DNA and DNA-bound histone proteins. In general, histone modifications are dynamic and include acetylation, methylation, isomerization, phosphorylation, sumoylation, and ubiquitination [1, 2]. The combination of such modifications confers enormous flexibility in terms of functional response of an individual cell towards extracellular signals and environmental stimuli. Certain modifications such as histone methylation can display additional layers of complexity regarding their methylation degree and undergo mono-, di-, or tri-methylation of lysine residues [2, 3]. Furthermore, combinations or sequential additions of different histone marks can affect the chromatin organization and subsequently alter the expression of the corresponding target genes [4]. Conventionally, DNA methylation was considered to be a stable epigenetic mark, although this notion is being challenged by recent reports of active DNA demethylation [5]. In mammals, DNA methylation strictly happens at the cytosine residues in the context of CpG dinucleotides. The methylation of DNA molecules is processed by a group of enzymes called DNA methyltransferases (DNMTs). The mammalian DNMT family consists of 5 proteins (DNMT1, 2, 3A, 3B, 3L). DNMT1 is involved in maintaining the DNA methylation pattern during replication, while DNMT3A and DNMT3B act as de novo methyltransferases. DNMT3L is essential for the establishment of maternal genomic imprints during oocyte development, and DNMT2 is classified as part of the DNMT family; however it has very weak catalytic activity [6].
DNA methylation is often associated with transcriptional repression and has been linked to the tissue-specific regulation of genes [7], expression of imprinted genes [8], and X-chromosome inactivation in females [9]. In general, DNA methylation affects gene expression in two ways: (i) directly, by altering the binding sites of transcription factors, or (ii) indirectly, via recruitment of proteins that recognize and bind to the methylated DNA and in turn modulate gene expression. The first group of proteins that were discovered with the potential of binding to methylated DNA were the MBD (methyl-binding domain) protein family members. The mammalian MBD family consists of 5 nuclear proteins, MBD 1–4 and MeCP2 (Methyl CpG binding protein 2). With the exception of MBD3, all MBD proteins share a conserved methyl-binding domain, through which they bind to methylated DNA [10]. MBD3 lacks such ability due to a critical mutation in its MBD domain [11]. MECP2 is an X-linked gene, which was discovered as the prototype member of the DNA methyl binding proteins (MBPs) [12]. Mutations in MECP2 are the primary cause of Rett Syndrome (RTT), a neurological disorder predominantly affecting young females. RTT is characterized by an apparently normal development for the first 6–18 months after birth, followed by regression of acquired motor and language skills [13–15]. In addition to Rett Syndrome, mutations in MECP2 have been observed in patients with classical autism, neonatal encephalopathy, and X-linked mental retardation [16–19].
Studies on MeCP2 have yielded surprising results in terms of the diversity of its functions (Figure 1) with enormous potential for epigenetic regulation of target gene expression. MeCP2 was initially identified as a methyl-binding protein [20]. Further investigations on MeCP2 function led to the discovery of its role as a transcriptional repressor and association with corepressor complexes such as mSin3A and HDACs [21, 22]. This was not surprising, since DNA methylation itself was considered to be a repressive mark. However, a genomewide search for MeCP2 genomic distribution in SH-SY5Y cells led to two surprising observations: (i) MeCP2 was found to be associated often with transcriptionally active genes; (ii) only 2.2% of the most methylated promoters were bound by MeCP2. The presence of MeCP2 at the active promoters was later observed in mouse hypothalamus, where MeCP2 was observed to be bound to approximately 85% of genes which were misregulated by overexpression or absence of MeCP2 [23]. These studies highlight the many facets of MeCP2 functions and emphasize the need to further study its known functions. In this review, we will discuss the role of MeCP2 in chromatin structure and nuclear architecture of neurons, its competition with the linker histone H1, the MECP2 transcript products and diverse functional domains of MeCP2 protein, as well as MeCP2 expression and genomic targets in neurons and glia.
https://www.hindawi.com/journals/np/2012/415825/
Free Radic Biol Med. 2015 Nov;88(Pt A):81-90. doi: 10.1016/j.freeradbiomed.2015.04.019. Epub 2015 May 8.
Exploring the possible link between MeCP2 and oxidative stress in Rett syndrome.
Filosa S1, Pecorelli A2, D'Esposito M3, Valacchi G4, Hajek J2.
Author information
Abstract
Rett syndrome (RTT, MIM 312750) is a rare and orphan progressive neurodevelopmental disorder affecting girls almost exclusively, with a frequency of 1/15,000 live births of girls. The disease is characterized by a period of 6 to 18 months of apparently normal neurodevelopment, followed by early neurological regression, with a progressive loss of acquired cognitive, social, and motor skills. RTT is known to be caused in 95% of the cases by sporadic de novo loss-of-function mutations in the X-linked methyl-CpG-binding protein 2 (MECP2) gene encoding methyl-CpG binding protein 2 (MeCP2), a nuclear protein able to regulate gene expression. Despite almost two decades of research into the functions and role of MeCP2, little is known about the mechanisms leading from MECP2 mutation to the disease. Oxidative stress (OS) is involved in the pathogenic mechanisms of several neurodevelopmental and neurodegenerative disorders, although in many cases it is not clear whether OS is a cause or a consequence of the pathology. Fairly recently, the presence of a systemic OS has been demonstrated in RTT patients with a strong correlation with the patients' clinical status. The link between MECP2 mutation and the redox imbalance found in RTT is not clear. Animal studies have suggested a possible direct correlation between Mecp2 mutation and increased OS levels. In addition, the restoration of Mecp2 function in astrocytes significantly improves the developmental outcome of Mecp2-null mice and reexpression of Mecp2 gene in the brain of null mice restored oxidative damage, suggesting that Mecp2 loss of function can be involved in oxidative brain damage. Starting from the evidence that oxidative damage in the brain of Mecp2-null mice precedes the onset of symptoms, we evaluated whether, based on the current literature, the dysfunctions described in RTT could be a consequence or, in contrast, could be caused by OS. We also analyzed whether therapies that at least partially treated some RTT symptoms can play a role in defense against OS. At this stage we can propose that OS could be one of the main causes of the dysfunctions observed in RTT. In addition, the major part of the therapies recommended to alleviate RTT symptoms have been shown to interfere with oxidative homeostasis, suggesting that MeCP2 could somehow be involved in the protection of the brain from OS.
https://www.ncbi.nlm.nih.gov/pubmed/25960047
Rett syndrome: disruption of epigenetic control of postnatal neurological functions
Amy E. Pohodich1,4 and Huda Y. Zoghbi1,2,3,4,*
Author information Article notes Copyright and License information Disclaimer
This article has been cited by other articles in PMC.
Go to:
Abstract
Loss-of-function mutations in the X-linked gene Methyl-CpG-binding protein 2 (MECP2) cause a devastating pediatric neurological disorder called Rett syndrome. In males, these mutations typically result in severe neonatal encephalopathy and early lethality. On the other hand, owing to expression of the normal allele in ~50% of cells, females do not suffer encephalopathy but instead develop Rett syndrome. Typically females with Rett syndrome exhibit a delayed onset of neurologic dysfunction that manifests around the child's first birthday and progresses over the next few years. Features of this disorder include loss of acquired language and motor skills, intellectual impairment and hand stereotypies. The developmental regression observed in patients with Rett syndrome arises from altered neuronal function and is not the result of neurodegeneration. Maintenance of an appropriate level of MeCP2 appears integral to the function of healthy neurons as patients with increased levels of MeCP2, owing to duplication of the Xq28 region encompassing the MECP2 locus, also present with intellectual disability and progressive neurologic symptoms. Despite major efforts over the past two decades to elucidate the molecular functions of MeCP2, the mechanisms underlying the delayed appearance of symptoms remain unclear. In this review, we will highlight recent findings that have expanded our knowledge of MeCP2's functions, and we will discuss how epigenetic regulation, chromatin organization and circuit dynamics may contribute to the postnatal onset of Rett syndrome.
Link to full text:
https://www.ncbi.nlm.nih.gov/pmc/articles/PMC4571996/pdf/ddv217.pdf
Rett Syndrome and MeCP2: Linking Epigenetics and Neuronal Function
Mona D. Shahbazian1 and Huda Y. Zoghbi1,2,3,4,5
Introduction
Rett syndrome (RTT [MIM #312750]) was discovered when two girls who exhibited the same unusual behaviors happened to be seated next to each other in the waiting room of Andreas Rett, a Viennese pediatrician. It took >30 years after this discovery to determine the genetic basis of RTT, largely because the disease is primarily sporadic in nature and because familial cases are scarce. The discovery that mutations in methyl-CpG–binding protein 2 (MECP2) cause RTT and other neurodevelopmental disorders has called attention to the importance of epigenetic modifications in neuronal function.
Clinical Features
RTT affects ~1/15,000 females worldwide (Hagberg 1985; Kerr and Stephenson 1985; Kozinetz et al. 1993). The classic form of the disease, found almost exclusively in females, follows a distinct developmental course that Andreas Rett described as “both tragic and fascinating” (Rett 1986). Affected girls are born healthy, appear to develop normally until age 6–18 mo, and achieve the expected motor, language, and social milestones (Hagberg et al. 1983). Their neurological development is then arrested and begins regressing in a predictable pattern comprising roughly four stages (Hagberg and Witt-Engerstrom 1986). During Stage I (age 6–18 mo), girls cease to acquire new skills; they display decelerating head growth and autistic features such as emotional withdrawal and diminished eye contact. In Stage II (age 1–4 years), affected children lose learned skills such as speech and purposeful hand use. They develop irregular breathing patterns, truncal and gait ataxia/apraxia, and stereotypical hand wringing; about half the girls also develop seizures. There is some stabilization of the disease during Stage III (age 4–7 years), because loss of skills can continue only to a point. During this stage, girls may learn to communicate preferences using eye pointing. They still have gross cognitive and motor impairments and commonly experience epileptic episodes. Seizures become less frequent during Stage IV (age 5–15 years and older), but motor deterioration continues. Hypoactivity, especially among those who cannot walk, contributes to the frequent development of scoliosis, which can cause the girls to be confined to wheelchairs (Hagberg and Witt-Engerstrom 1986).
Other features are associated with RTT but are not diagnostic. For example, patients with RTT are generally small for their age (Holm 1986). Some have suggested that this growth retardation is due to poor self-feeding abilities and reduced caloric intake (Thommessen et al. 1992), but others have found it to be independent of diet (Rice and Haas 1988). Many patients display an abnormally long QTc interval (Sekul et al. 1994; Guideri et al. 1999). Although females with RTT often live well into adulthood, the mortality rate is 1.2% per year, with about 1/4 of these deaths described as sudden and unexplained (Kerr et al. 1997). Incidents of sudden death have been postulated to result from breathing dysfunction and cardiac abnormalities (Sekul et al. 1994; Guideri et al. 1999). Finally, patients with RTT often have signs of autonomic dysfunction such as constipation and cold or pale extremities (Hagberg et al. 1983; Naidu et al. 1986).
In addition to classic RTT (summarized in table 1), five distinct categories of atypical cases have been delineated on the basis of clinical criteria (Hagberg and Skjeldal 1994). These variants have some, but not all, diagnostic features of RTT and can be milder or more severe. Milder variants include the forme fruste (“worn-down form”), the late regression variant, and the preserved speech variant (Hagberg and Witt-Engerstrom 1986; Zappella 1992; Hagberg and Skjeldal 1994). The more severe forms are the congenital form and the early-seizure-onset variant (Hanefeld 1985; Goutieres and Aicardi 1986; Hagberg and Skjeldal 1994).
Link to full text:
https://www.ncbi.nlm.nih.gov/pmc/articles/PMC378559/pdf/AJHGv71p1259.pdf
Recent AVXL News
- Anavex Life Sciences to Present at the H.C. Wainwright 26th Annual Global Investment Conference 2024 • GlobeNewswire Inc. • 09/03/2024 11:30:00 AM
- Form 10-Q - Quarterly report [Sections 13 or 15(d)] • Edgar (US Regulatory) • 08/06/2024 09:21:05 PM
- Anavex Life Sciences Reports Fiscal 2024 Third Quarter Financial Results and Provides Business Update • GlobeNewswire Inc. • 08/06/2024 11:30:00 AM
- Anavex Life Sciences to Announce Fiscal 2024 Third Quarter Financial Results on Tuesday, August 6, 2024 • GlobeNewswire Inc. • 08/01/2024 11:30:00 AM
- Form 8-K - Current report • Edgar (US Regulatory) • 07/30/2024 09:20:53 PM
- Anavex Life Sciences Announces Translational Biomarker Data for ANAVEX®2-73 (blarcamesine) in Fragile X Syndrome (Major Cause of Autism) at the 19th NFXF International Fragile X Conference • GlobeNewswire Inc. • 07/30/2024 11:30:00 AM
- Form S-3 - Registration statement under Securities Act of 1933 • Edgar (US Regulatory) • 07/29/2024 09:21:49 PM
- Results from Anavex Life Sciences Landmark Phase IIb/III Trial of Blarcamesine Presented at Alzheimer's Association Conference • GlobeNewswire Inc. • 07/28/2024 09:00:00 PM
- Anavex Life Sciences to Present at the H.C. Wainwright 5th Annual Neuro Perspectives Virtual Conference • GlobeNewswire Inc. • 06/20/2024 11:30:00 AM
- Form 4 - Statement of changes in beneficial ownership of securities • Edgar (US Regulatory) • 06/17/2024 11:30:10 AM
- Anavex Life Sciences Announces Expansion of Leadership Team • GlobeNewswire Inc. • 05/22/2024 11:30:00 AM
- Form 4 - Statement of changes in beneficial ownership of securities • Edgar (US Regulatory) • 05/17/2024 10:01:00 AM
- Anavex Life Sciences to Present at the H.C. Wainwright 2nd BioConnect Investor Conference at NASDAQ • GlobeNewswire Inc. • 05/14/2024 11:30:00 AM
- Shareholders that lost money on Anavex Life Sciences Corporation(AVXL) Urged to Join Class Action - Contact The Gross Law Firm to Learn More • PR Newswire (US) • 05/10/2024 09:45:00 AM
- Form 10-Q - Quarterly report [Sections 13 or 15(d)] • Edgar (US Regulatory) • 05/09/2024 08:35:55 PM
- Form 8-K - Current report • Edgar (US Regulatory) • 05/09/2024 12:00:30 PM
- Anavex Life Sciences Reports Fiscal 2024 Second Quarter Financial Results and Provides Business Update • GlobeNewswire Inc. • 05/09/2024 11:30:00 AM
- The Gross Law Firm Announces the Filing of a Securities Class Action on Behalf of Anavex Life Sciences Corporation(AVXL) Shareholders • PR Newswire (US) • 05/07/2024 09:45:00 AM
- Form DEFA14A - Additional definitive proxy soliciting materials and Rule 14(a)(12) material • Edgar (US Regulatory) • 05/06/2024 10:03:33 AM
- Anavex Life Sciences Corporation Sued for Securities Law Violations - Contact The Gross Law Firm Before May 13, 2024 to Discuss Your Rights - AVXL • PR Newswire (US) • 05/03/2024 09:45:00 AM
- Anavex Life Sciences to Announce Fiscal 2024 Second Quarter Financial Results on Thursday, May 9th, 2024 • GlobeNewswire Inc. • 05/02/2024 11:30:00 AM
- May 13, 2024 Deadline: Contact The Gross Law Firm to Join Class Action Suit Against AVXL • PR Newswire (US) • 04/26/2024 09:45:00 AM
- Contact The Gross Law Firm by May 13, 2024 Deadline to Join Class Action Against Anavex Life Sciences Corporation(AVXL) • PR Newswire (US) • 04/19/2024 09:45:00 AM
- The Gross Law Firm Reminds Shareholders of a Lead Plaintiff Deadline of May 13, 2024 in Anavex Life Sciences Lawsuit - AVXL • PR Newswire (US) • 04/16/2024 09:45:00 AM
North Bay Resources Commences Operations at Bishop Gold Mill, Inyo County, California; Engages Sabean Group Management Consulting • NBRI • Sep 25, 2024 9:15 AM
CEO David B. Dorwart Anticipates a Bright Future at Good Gaming Inc. Through His Most Recent Shareholder Update • GMER • Sep 25, 2024 8:30 AM
Cannabix Technologies and Omega Laboratories Inc. Advance Marijuana Breathalyzer Technology - Dr. Bruce Goldberger to Present at Society of Forensic Toxicologists Conference • BLOZF • Sep 24, 2024 8:50 AM
Integrated Ventures, Inc Announces Strategic Partnership For GLP-1 (Semaglutide) Procurement Through MedWell USA, LLC. • INTV • Sep 24, 2024 8:45 AM
Avant Technologies Accelerates Creation of AI-Powered Platform to Revolutionize Patient Care • AVAI • Sep 24, 2024 8:00 AM
VHAI - Vocodia Partners with Leading Political Super PACs to Revolutionize Fundraising Efforts • VHAI • Sep 19, 2024 11:48 AM