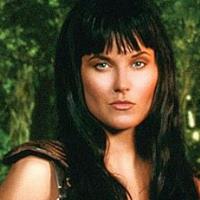
Monday, December 03, 2018 10:25:54 AM
Restoration of sleep may be the key to stopping the advance of Alzheimer's in the preclinical stage.
1. Amyloid-b and Alzheimer’s disease pathogenesis Alzheimer’s disease (AD) is a neurodegenerative disorder characterized by progressive cognitive impairment that is a current and growing public health crisis that only has minimally effective treatments. In 2010, more than 5 million Americans aged 65 years were living with AD, and this number is expected to increase to 13.5 million in 2050 (Alzheimer’s Association, 2010). A reliable, even modest reduction in the risk of AD would have a tremendous public health impact. Age is the greatest risk factor for AD, but the progression to cognitive impairment likely results from genetic (e.g., ApoE4) and environmental (e.g., exercise, diet, and sleep) factors that influence AD pathology (Mayeux and Stern, 2012). Recent evidence in both humans and animal models suggests a possible mechanism through which sleep, likely interacting with other genetic and environmental risk factors, may play a role in AD pathogenesis.
Deposition of extracellular amyloid-b (Ab) into insoluble plaques in the brain is a key early step in the pathogenesis of AD that is associated with the aggregation of tau into intracellular neurofibrillary tangles, synaptic dysfunction, neuronal loss, and cognitive impairment (Hardy and Selkoe, 2002; Jack et al., 2010). The Ab peptide is predominantly produced in the brain by neurons when amyloid precursor protein (APP) is cleaved by b- and gsecretases into multiple isoforms of different amino acid lengths (Strooper et al., 2010). More specifically, g-secretase cuts the C-terminal end of the Ab peptide to generate 3 major isoforms, that is, Ab38, Ab40, and Ab42. Ab isoforms are then secreted into the interstitial fluid (ISF) through synaptic vesicle exocytosis, which is a process influenced by synaptic activity (Cirrito et al., 2005). The contribution of Ab isoforms to plaque formation varies. Whereas Ab40 is produced at higher concentrations, Ab42 is more hydrophobic, neurotoxic, and prone to aggregate (Jarrett et al., 1993). Further, the aggregation of Ab into extracellular plaques has been found to be concentration dependent. In a mouse model study, neural cells were transplanted from APP23 transgenic mice into wild-type mice and Ab levels were measured both within the neural graft and the surrounding ISF. Ab concentrations were the highest within the graft and decreased as a function of the distance from the graft, and a similar gradient pattern was observed for amyloid plaques (Meyer-Luehmann et al., 2003). Since all the Ab deposition did not occur only within the grafts, it was suggested that Ab diffusion into surrounding neural tissue played a role in plaque formation.
Neuropathologic changes in AD, that is, extracellular amyloid plaques and intracellular neurofibrillary tangles of aggregatedtau, likely proceed in a sequential anatomic pattern involving the entorhinal cortex, hippocampus, and medial temporal lobe (Braak and Braak, 1991). Ab deposition is probably insufficient to result in cognitive decline because pathologic studies show that significant neuronal and synaptic loss has already occurred by the time clinical symptoms manifest. Further, amyloid deposition can be assessed in vivo in humans by positron emission tomography (PET) with an amyloid tracer, that is, Pittsburgh Compound B (PiB) that binds to amyloid, or by decreased cerebrospinal fluid (CSF) Ab42 concentrations. Approximately 25%e30% of individuals in their eight decade who were cognitively normal that were assessed by either of these methods have been found to have amyloid deposition (Mintun et al., 2006; Morris et al., 2009). The period of amyloid deposition with normal cognitive functioning has been described as “preclinical Alzheimer’s disease” (Jack et al., 2010; Sperling et al., 2011). Treatment of AD is hypothesized to be the most successful in this preclinical phase before Ab deposition into Ab-containing plaques and tau aggregation occurs, which causes significant cell loss leading to the onset of clinically detectable cognitive impairment (Morris, 2005).
Amyloid imaging has also shown that Ab deposition co-localizes anatomically with other imaging abnormalities associated with AD in many regions of the brain. Abnormalities that co-localize with PiB-PET include brain atrophy as shown on brain magnetic resonance imaging, hypometabolism measured by fluorodeoxyglucosePET, and dysfunction of the default mode network (DMN) as measured by functional magnetic resonance imaging (Bero et al., 2011; Buckner et al., 2005). The DMN is the resting state network most active in the absence of task performance (Raichle et al., 2000) and has been reported as the first network affected by AD (Greicius et al., 2004). The DMN is associated with episodic memory (Buckner, 2004), which is a cognitive domain that is impaired early in AD. Since the DMN is a resting state network and is therefore synaptically and metabolically more active than other regions of the brain, this co-localization with AD pathology may be because of increased Ab production and secretion into the ISF resulting in a higher local concentration and greater deposition. Additionally, decreased energy metabolism and atrophy in the brain have also been correlated with areas of amyloid deposition (Vlassenko et al., 2010). Because all these imaging abnormalities overlap, these findings suggest that increased synaptic activity leading to Ab aggregation into plaques progresses to decreased neuronal metabolic function and atrophy presumably through neuronal loss. This hypothesis is supported by findings in the dominantly inherited AD population (Bateman et al., 2012).
2. Sleep and AD Sleep serves a restorative function in the brain and is involved with memory retention. More specifically, slow-wave sleep (SWS) plays a critical role in the consolidation of long-term memory (Born and Wilhelm, 2012). Good quality sleep involves following a day and/or night (i.e., diurnal) pattern of alertness and activity during the day followed by quiescence at night. Cycling several times through the different sleep stages during the sleep period is also essential to restorative sleep. Scoring an individual as awake or in a specific sleep stage is primarily determined by changes on an electroencephalogram (EEG) recorded during polysomnography. In the awake state, the EEG shows low amplitude, high-frequency fluctuations because of neurons in the cerebral cortex firing irregularly. As wakefulness gives way to sleep, the low amplitude, highfrequency activity attenuates as cortical neurons undergo a slow oscillation (<1 Hz) in membrane potential between a hyperpolarized state with no neuronal firing to a depolarized state of intense firing (Massimini et al., 2004). This slow oscillation is the fundamental cellular process that organizes waveforms seen on EEG during sleep, that is, sleep spindles and slow waves.
There are 4 sleep stages. The EEG background in rapid eye movement sleep is similar to the awake state with low amplitude, mixed-frequency activity. Non-rapid eye movement (NREM) sleep is characterized by 3 stages that exhibit progressively increased slow wave activity (SWA): N1 or drowsiness, N2, and N3 or SWS. During the deeper stages of NREM sleep (N2 and N3) there are more high-amplitude slow waves, which likely accounts for the general decrease in regional synaptic activity during NREM sleep (Vyazovskiy et al., 2011). These periods of deeper NREM sleep with increased SWA are hypothesized to decrease synaptic strength to a level that is energetically sustainable and promotion of synaptic plasticity and memory (Tononi and Cirelli, 2006).
Nearly all people older than the age of 60 years have disrupted sleep architecture and decreased SWS (Redline et al., 2004). Further, aging has also been associated with regional brain atrophy involving the midline frontal lobe regions (Sowell et al., 2003) and cognitive decline (Buckner, 2004). These 3 factors have been independently associated with aging. However, a recent study found that age-related medial prefrontal cortex gray-matter atrophy was associated with reduced NREM SWA in older adults, the extent of which statistically accounted for the impairment of overnight sleep-dependent memory retention (Mander et al., 2013). These recent findings suggest an additional potential mechanism that may be linked to the sleep changes that have been observed in aging that may be contributing to cognitive decline in older individuals.
Sleep disturbances in individuals with AD are multifaceted and include increased or decreased total sleep time, nocturnal arousals, and reversal of the day and/or night sleep pattern (McCurry et al., 1999). However, these sleep disturbances have been measured in individuals already exhibiting cognitive impairment and are likely a manifestation of dementia. Sleep interventions at this stage of AD may be difficult to both implement and achieve positive benefits. For example, the incidence of sleep disorders (e.g., sleep apnea) is increased in patients with AD and continuous positive airway pressure (CPAP) therapy may slow or improve cognitive functioning in patients with AD and sleep disordered breathing (SDB) (AncoliIsrael et al., 2008; Cooke et al., 2009). However, both the diagnosis and treatment of sleep disorders (e.g., SDB) in patients with AD is challenging because of the patients’ underlying cognitive dysfunction impeding both the diagnosis via polysomnography and treatment with CPAP. Therefore, the efficacy of current therapies (e.g., CPAP) is difficult to assess in an AD patient population (Yesavage et al., 2003).
Recent research has focused on the risk of developing cognitive impairment in cognitively-normal individuals with long-term sleep disturbances. In multiple cross-sectional studies, changes in sleep duration have been associated with an increased risk of cognitive impairment. Tworoger et al. (2006) observed that selfreported difficulty sleeping and sleep duration 5 hours/night in older women was associated with poorer cognitive performance for general cognition, verbal memory, category fluency, and attention. Others have shown conflicting results including cognitive impairment associated with longer sleep times >11 hours/ night (Faubel et al., 2009) or 9 hours/night (Loerbroks et al., 2010), but not short sleep time or both short and long sleep time (Ferrie et al., 2011; Kronholm et al., 2009; Xu et al., 2011). Additional markers of poor sleep quality, that is, low sleep efficiency, prolonged sleep latency, increased wake after sleep onset, and increased napping, have all been associated with impaired cognitive function (Blackwell et al., 2006, 2011; Keage et al., 2012; Potvin et al., 2012).
4. Sleep, Ab, and Alzheimer’s disease pathophysiology: a proposed model
The diurnal Ab pattern is hypothesized to be related to higher neuronal activity during wakefulness and decreased neuronal activity during sleep, such as occurs in SWS (Nir et al., 2011). Synaptic activity has been shown to increase ISF Ab release from neurons in both the mouse and in humans (Brody et al., 2008; Cirrito et al., 2005). Loss of this diurnal pattern is likely because of sequestration of Ab in extracellular amyloid plaques (e.g., altered clearance) or altered neuronal firing (e.g., altered production). There is evidence to support Ab42 sequestration in plaques in older individuals with amyloid deposition that leads to decreased clearance and lower, less variable CSF Ab42 concentrations. Production of soluble Ab may be relatively increased during the sleep period by loss of SWS in the context of aging and/or sleep disturbances such as sleep apnea or insomnia. The net effect of these sleep changes is to increase wake time during the sleep period. More specifically, the DMN deactivates during sleep, particularly SWS (Samann et al., 2011). Therefore,decreased sleep efficiency and decreased SWS will lead to a lack of deactivation of the DMN, relative increases in synaptic and metabolic neuronal activity, increased soluble CSF Ab levels during the sleep period, increased Ab aggregation and sequestration into plaques, and attenuation of the Ab diurnal pattern.
Although changes in the sleep-wake cycle have been associated with markers of AD pathology such as amyloid deposition, the timing, role, and extent of these changes that are associated with increasing stages of AD neuropathology and cognitive dysfunction is unclear. Findings in animal models hint that driving Ab concentrations up or down with sleep deprivation or sleep induction, respectively, may affect amyloid aggregation into plaques. This finding has not been replicated in humans, but changes of Ab production by 25%e40% (Jonsson et al., 2012) can completely protect or cause AD in humans (Jonghe et al., 1999), which suggests that increasing SWS time may decrease or prevent Ab accumulation. Notably, a 25% change in Ab concentration has been found between wakefulness and sleep (Huang et al., 2012). An alternative explanation of the current data is that sleep changes are a marker for progression of AD pathology rather than a key event in AD pathogenesis. Further research is needed to differentiate between these hypotheses.
A model for the role of sleep in AD pathogenesis is proposed to test future hypothesis-driven research (Fig. 2). As previously discussed, there are numerous changes that occur during aging including regional brain atrophy (e.g., medial prefrontal cortex) and changes in sleep parameters, that is, increased wake after sleep onset, decreased sleep efficiency, decreased SWS, and an increased incidence of sleep disorders (e.g., SDB). These changes occur in individuals with genetic and environmental risk factors that affect overall risk of AD. In individuals prone to AD based on these risk factors, sleep may play a more or less significant role in the development of AD.
The net effect of disturbed sleep parameters in older adults is a relative increase in synaptic and metabolic activity in the brain during the sleep period compared with younger individuals. Therefore, the concentration of Ab in the CSF does not decrease during sleep as expected and results in attenuation of the Ab diurnal pattern. Ab concentrations during the sleep period are maintained at relatively high levels (25% higher than during sleep), which promotes amyloid deposition that may further feedback to disturb sleep and elevate Ab levels during sleep.
The regions of the brain most at risk for amyloid deposition in this proposed model are those that are metabolically most active, that is, the DMN. Ab deposition in brain regions is important because the DMN leads to network dysfunction, synaptic dysfunction, and impaired cognitive functioning. Impaired cognitive functioning and network dysfunction will manifest as hypometabolism or vice versa. Impaired cognitive function is associated with sleep disturbances, feeding back to the sleep changes associated with aging. The net effect is to increase Ab deposition that is a known marker for AD risk.
Link to the complete paper:
https://www.sciencedirect.com/science/article/pii/S0197458014003509?via%3Dihub
Recent AVXL News
- Anavex Life Sciences Corporation Sued for Securities Law Violations - Contact The Gross Law Firm Before May 13, 2024 to Discuss Your Rights - AVXL • PR Newswire (US) • 05/03/2024 09:45:00 AM
- Anavex Life Sciences to Announce Fiscal 2024 Second Quarter Financial Results on Thursday, May 9th, 2024 • GlobeNewswire Inc. • 05/02/2024 11:30:00 AM
- May 13, 2024 Deadline: Contact The Gross Law Firm to Join Class Action Suit Against AVXL • PR Newswire (US) • 04/26/2024 09:45:00 AM
- Contact The Gross Law Firm by May 13, 2024 Deadline to Join Class Action Against Anavex Life Sciences Corporation(AVXL) • PR Newswire (US) • 04/19/2024 09:45:00 AM
- The Gross Law Firm Reminds Shareholders of a Lead Plaintiff Deadline of May 13, 2024 in Anavex Life Sciences Lawsuit - AVXL • PR Newswire (US) • 04/16/2024 09:45:00 AM
- Class Action Filed Against Anavex Life Sciences Corporation (AVXL) - May 13, 2024 Deadline to Join - Contact The Gross Law Firm • PR Newswire (US) • 04/12/2024 09:45:00 AM
- Anavex Life Sciences to Present at the Noble Capital Markets Virtual Healthcare Equity Conference • GlobeNewswire Inc. • 04/11/2024 11:30:00 AM
- Class Action Filed Against Anavex Life Sciences Corporation (AVXL) - May 13, 2024 Deadline to Join - Contact The Gross Law Firm • PR Newswire (US) • 04/09/2024 09:45:00 AM
- Anavex Life Sciences Corporation Class Action: The Gross Law Firm Reminds Anavex Life Sciences Investors of the Pending Class Action Lawsuit with a Lead Plaintiff Deadline of May 13, 2024 - AVXL • PR Newswire (US) • 04/05/2024 09:45:00 AM
- Shareholders that lost money on Anavex Life Sciences Corporation(AVXL) should contact The Gross Law Firm about pending Class Action - AVXL • PR Newswire (US) • 04/02/2024 09:45:00 AM
- Lost Money on Anavex Life Sciences Corporation(AVXL)? Join Class Action Suit Seeking Recovery - Contact The Gross Law Firm • PR Newswire (US) • 03/29/2024 09:45:00 AM
- Investors who lost money on Anavex Life Sciences Corporation(AVXL) should contact The Gross Law Firm about pending Class Action - AVXL • PR Newswire (US) • 03/26/2024 09:45:00 AM
- Anavex Life Sciences to Present at the 23rd Annual Needham Virtual Healthcare Conference • GlobeNewswire Inc. • 03/25/2024 11:30:00 AM
- The Gross Law Firm Notifies Anavex Life Sciences Corporation Investors of a Class Action Lawsuit and Upcoming Deadline • PR Newswire (US) • 03/22/2024 09:45:00 AM
- Anavex Life Sciences Initiates Placebo-Controlled U.S. Phase 2 Clinical Trial of ANAVEX®3-71 in Schizophrenia • GlobeNewswire Inc. • 03/18/2024 11:30:00 AM
- Anavex Life Sciences to Present at the 44th Annual TD Cowen Health Care Conference • GlobeNewswire Inc. • 02/26/2024 12:30:00 PM
- Form 4 - Statement of changes in beneficial ownership of securities • Edgar (US Regulatory) • 02/23/2024 11:05:18 AM
- Form 4 - Statement of changes in beneficial ownership of securities • Edgar (US Regulatory) • 02/23/2024 11:04:32 AM
- Form 4 - Statement of changes in beneficial ownership of securities • Edgar (US Regulatory) • 02/23/2024 11:04:31 AM
- Form 4 - Statement of changes in beneficial ownership of securities • Edgar (US Regulatory) • 02/23/2024 11:03:48 AM
- Form 4 - Statement of changes in beneficial ownership of securities • Edgar (US Regulatory) • 02/23/2024 11:03:06 AM
- Form 10-Q - Quarterly report [Sections 13 or 15(d)] • Edgar (US Regulatory) • 02/07/2024 09:31:07 PM
- Anavex Life Sciences Reports Fiscal 2024 First Quarter Financial Results and Provides Business Update • GlobeNewswire Inc. • 02/07/2024 12:30:00 PM
- Anavex Life Sciences to Announce Fiscal 2024 First Quarter Financial Results on Wednesday, February 7th, 2024 • GlobeNewswire Inc. • 02/01/2024 12:30:00 PM
- Form SC 13G/A - Statement of acquisition of beneficial ownership by individuals: [Amend] • Edgar (US Regulatory) • 01/25/2024 10:01:34 PM
NanoViricides Reports that the Phase I NV-387 Clinical Trial is Completed Successfully and Data Lock is Expected Soon • NNVC • May 2, 2024 10:07 AM
ILUS Files Form 10-K and Provides Shareholder Update • ILUS • May 2, 2024 8:52 AM
Avant Technologies Names New CEO Following Acquisition of Healthcare Technology and Data Integration Firm • AVAI • May 2, 2024 8:00 AM
Bantec Engaged in a Letter of Intent to Acquire a Small New Jersey Based Manufacturing Company • BANT • May 1, 2024 10:00 AM
Cannabix Technologies to Deliver Breath Logix Alcohol Screening Device to Australia • BLO • Apr 30, 2024 8:53 AM
Hydromer, Inc. Reports Preliminary Unaudited Financial Results for First Quarter 2024 • HYDI • Apr 29, 2024 9:10 AM