
Monday, September 05, 2011 5:15:27 AM
Why Life Originated (And Why it Continues)
One of the earliest known forms of life on Earth is stromatolites, which consist of cyanobacteria (blue-green algae). Fossil records of stromatolites date back 3.5 billion years. This photo shows modern stromatolites in Shark Bay, Western Australia.
Image credit: Paul Harrison.
By Lisa Zyga
December 9, 2008
(PhysOrg.com) -- Today, scientists understand pretty well how life evolves, by mechanisms based on Darwin’s theory of natural selection for survival of the fittest. However, Darwin’s 1859 classic, On the Origin of Species, somewhat ironically doesn’t answer that very question – how species actually originated. And to this day, how that first tiny pool of chemicals twitched to life remains a puzzle.
In a recent study called “Why did life emerge?”, two scientists, son and father Arto Annila of the University of Helsinki and Erkki Annila of the Finnish Forest Research Institute, offer some insight into the general driving force of life’s origins in terms of thermodynamics. As they explain, all organisms are composed of molecules that assemble together via numerous chemical reactions. Just as heat flows from hot to cold, these molecules obey the universal tendency to diminish energy differences, so that the most likely chemical reactions are those in which energy flows “downhill” toward a stationary state, or chemical equilibrium.
Although the researchers don’t speculate on the specific chemical reactions that created life, they explain that the molecules involved most likely underwent a series of more and more complex reactions to minimize mutual energy differences between matter on Earth and with respect to high-energy radiation from Sun. The process eventually advanced so far that it cumulated into such sophisticated functional structures that could be called living.
“The most important idea in our study is that there is no distinction between animate and inanimate,” Arto Annila told PhysOrg.com. “Processes of life are, in their principles, no different from any other natural processes.”
In their study, which is published in the International Journal of Astrobiology, the researchers considered a primordial pool that contained some basic compounds. By reacting with one another and coupling with an external energy source such as the Sun, the compounds formed a chemical system. The compounds continually engaged in chemical reactions, thriving the most when capturing and distributing more and more of the Sun’s energy in the quest for a steady state. The evolutionary process was and still is non-deterministic, even chaotic, since the energy flows create energy differences that in turn affect the flows.
Due to random variations stemming from the chemical reactions, some novel compounds may have emerged in the primordial system. Some of these compounds (such as those involving carbon) might have been exceptionally good at creating energy flow, enabling the system to diminish energy differences very efficiently and reach a higher level of entropy. Compounds with these advantages would have gained ground during this period of primitive chemical evolution. But the scientists emphasize that identifying which exact compounds were key players during this period would be very difficult to determine.
“Today we may have only very little evidence left from the courses in the very distant past to deduce which chemical species went extinct, while others, more viable in energy transduction, emerged,” Arto Annila explained. In other words, this study focuses on why life emerged, not how.
What is more relevant, the scientists note, is the fact that the physical tendency to diminish energy differences makes no distinction between systems that are inanimate or animate. As the researchers explain, the order and complexity that characterize modern biological systems have no value in and of themselves, but structure and hierarchical organization emerged and developed because they provided paths for increasing energy flows.
The scientists give several examples of mechanisms associated with life that increase entropy. For instance, when systems (e.g. molecules) become entities of larger systems (e.g. cells) that participate in larger ranges of interactions to consume more free energy, entropy increases. Genetic code might have served as another primordial mechanism, acting as a catalyst that could increase energy flow toward greater entropy. Today, complex organisms have cellular metabolism, which is another mechanism that increases entropy, as it disperses energy throughout the organism and into the environment. The food chain in an ecosystem is another example of a mechanism for transferring energy on a larger scale.
In this sense, life is a very natural thing, which emerged simply to satisfy basic physical laws. Our “purpose,” so to speak, is to redistribute energy on the Earth, which is in between a huge potential energy difference caused by the hot Sun and cold space. Organisms evolve via natural selection, but at the most basic level, natural selection is driven by the same thermodynamic principle: increasing entropy and decreasing energy differences. The natural processes from which life emerged, then, are the same processes that keep life going – and they operate on all timescales.
“According to thermodynamics, there was no striking moment or no single specific locus for life to originate, but the natural process has been advancing by a long sequence of steps via numerous mechanisms so far reaching a specific meaning – life,” the researchers explained.
And because thermodynamics recognizes no specific moment, particular place, compound or reaction that would distinguish animate from inanimate, a search for ‘the birth of life’ seems like an ill-posed project, Arto Annila explained.
“Indeed, the quest for the origin of life seems a futile endeavor because life in its entirety is a natural process that has, according to the second law of thermodynamics, no definite beginning,” he said. “To ask how life started would be the same as to ask when and where did the first wind blow that quivered the surface of a warm pond.”
More information: Annila, Arto and Annila, Erkki. “Why did life emerge?” International Journal of Astrobiology 7 (3 & 4): 293-300 (2008) [abstract http://journals.cambridge.org/action/displayAbstract?fromPage=online&aid=2820924 and http://arxiv.org/abs/0910.2621 , full text pdf http://www.helsinki.fi/~aannila/arto/whylife.pdf and http://arxiv.org/ftp/arxiv/papers/0910/0910.2621.pdf ].
Copyright 2008 PhysOrg.com.
http://www.physorg.com/news148050302.html [with comments]
===
A Marine Chemist Studies How Life Began
Jeffrey L. Bada, 67, is the distinguished professor of marine chemistry at the University of California, San Diego. He studies how life began.
Robert Benson for The New York Times
A Conversation With Jeffrey L. Bada
By CLAUDIA DREIFUS
Published: May 17, 2010
Jeffrey L. Bada, 67, is the distinguished professor of marine chemistry at the University of California, San Diego. He studies how life began. We spoke for an hour during the American Association for the Advancement of Science’s annual meeting in San Diego last winter and again this month by telephone. An edited version of the two conversations follows:
Q. HAVE YOU ALWAYS BEEN INTERESTED IN THE CHEMISTRY OF LIFE?
A. No. When I started in graduate school in 1965, I had ideas about becoming a theoretical chemist, applying quantum mechanics to chemistry. But when I arrived at U.C.S.D., I met Stanley Miller, who’d been a student of Nobel Prize-winning chemist Harold Urey. In 1953, they completed the classic experiment on the chemical origins of life. They’d taken gases present on the early Earth like methane, ammonia and hydrogen and applied a spark discharge to them, to mimic lightning. From that, they produced amino acids, the compounds that make up the proteins in all living organisms. It was a stunning discovery. So when I met Stanley, I was hooked. I switched my thesis to work with him. My Ph.D. idea was to move the spark discharge experiment a step forward by studying amino acid stability.
Q. WHY WAS STABILITY IMPORTANT TO UNDERSTAND?
A. Because, in the turbulent environment of the early Earth, if an amino acid had been too unstable it would have decomposed. There would have never been enough of it to make up what we call “the prebiotic soup,” the molecular ingredients of life. So I suggested this one set of reactions that might constrain their decomposition, and then, in the lab, we did an experiment, which worked the first time we tried it. We were able to show some of the conditions that would allow amino acids to exist for longer time periods. And this allowed us to understand what types of amino acids may have been present on the baby Earth.
Q. DO WE KNOW HOW THEY BECAME LIFE?
A. We are closing in on that question. The Earth had to cool down enough for water to appear. Water allows molecules to dissolve and interact, which is why it is essential to life. We do know that we went from simple molecules to more complex molecules and eventually to RNA, which evolved into DNA. This took about a billion years.
The missing piece of the puzzle is that intermediate phase between the amino acids and the RNA phase. We know that RNA is too complex to have arisen out of the simple molecules of the primordial soup. We can surmise that this intermediate form was able to make copies of itself to pass onto the next generation. Over time, mutations occurred and those mutants with survival advantages thrived and on and on until you eventually got to the complex RNA world.
Q. IS THIS SAME PROCESS AT WORK SOMEWHERE IN THE UNIVERSE NOW?
A. The chemistry we see in the lab is universal chemistry. It takes place anywhere you have the ingredients and conditions. We know that it happened, partially, on some of the meteorites that have come to earth. Amino acids have been found inside some of them.
Q. SO WE’RE PROBABLY NOT ALONE?
A. I don’t want to say “we” because people immediately think of something like a human being. But life as we know it — a self-replicating system — is probably not unique to the Earth. Under the right conditions, with the right chemistry, it can happen. There may be simple chemistry happening on Titan, a moon of Saturn. Some people think it could be happening on the satellites of Jupiter. There are compelling reasons to think that Mars was wet when it was young and that the raw materials for life could have been there. We don’t know how far it progressed.
Q. DID YOU SUPPORT FORMER PRESIDENT GEORGE W. BUSH’S PLAN FOR MANNED TRAVEL TO MARS?
A. I’m glad the Obama administration appears to be canceling it. Until we know whether or not life existed on Mars, putting humans there is premature. We’d contaminate the planet. All we’d see is human microbes and refuse.
Q. ON THE OTHER HAND, STEPHEN HAWKING THINKS EXPOSURE TO EXTRATERRESTRIAL LIFE COULD PROVE HAZARDOUS TO EARTHLINGS. “IF ALIENS VISIT US, THE OUTCOME WOULD BE MUCH AS WHEN COLUMBUS LANDED IN AMERICA, WHICH DIDN’T TURN OUT WELL FOR THE NATIVE AMERICANS,” DR. HAWKING RECENTLY WARNED. IS HE ONTO SOMETHING?
A. Dr. Hawking suggests an intriguing possibility, although there are alternative scenarios. Aliens might bring some exotic organisms with them to Earth, but would they survive in our oxygen-rich environment long enough to do any damage to humans or the ecosystem? Meteorites from Mars have landed on Earth throughout history, and if any Martian organisms were hitchhiking on these, they have not killed us yet.
Q. THREE YEARS AGO, YOU REDID THE SPARK DISCHARGE EXPERIMENT. WHY?
A. Actually, we didn’t redo it — we reanalyzed it. When, in 1999, Stanley had a stroke, he donated everything in his office to my laboratory. Eight years later, I was giving a talk in Texas and someone there told me that he’d once seen the extracts of the 1953 experiment in a cardboard box in Stanley’s laboratory. Stanley had kept them for all those decades! Though we were very close, he’d never told me about it. I suppose it was just old business to him. But when I got back to San Diego, I asked my staff, “When we moved everything from Stanley’s office, did we get a little cardboard box?” And someone said, “Yeah, its right over there.”
And there it was! Inside, were all these tiny glass vials carefully labeled, with page numbers referring Stanley’s laboratory notes. I was dumbstruck. We were looking at history. It immediately hit me that when Stanley had first done the experiment, analytic tools were still very, very primitive. We have instruments today that are a billion times better. So we then reanalyzed the original materials with the modern tools. And lo and behold, we found that the spark discharge experiment had actually made about 30 compounds. Stanley had shown they’d made only five!
Q. DID YOUR ANALYSIS CONFIRM THE VALIDITY OF THE EXPERIMENT? THERE ARE SOME PEOPLE WHO STILL DOUBT IT.
A. Oh, yes. And more! It showed how easy it was to make a huge number of compounds. What was really interesting was the Murchison meteorite [ http://www.scientificamerican.com/article.cfm?id=murchison-meteorite ], which fell to earth in 1969, was originally thought to contain maybe 75 amino acids. Three years ago, when we compared the distribution of amino acids in the discharge experiment, they were uncannily similar to those in the meteorite.
I would have loved to have told Stanley about this. But sadly, he was completely incapacitated by the time we found the samples. He died shortly thereafter. I would have loved to have said to him, “Look Stanley, your experiment duplicated what happened on Murchison and you didn’t know it.”
Q. DO YOU THINK IT WOULD BE VALUABLE FOR OTHER SCIENTISTS TO REVISIT OLDER EXPERIMENTS?
A. Listen, I was very fortunate to have had a mentor who had the foresight to preserve his experimental materials. Nonetheless, he couldn’t anticipate all the changes that came with modern analytical chemistry. Today, we are seeing an explosion of knowledge due to new techniques. We ought to use them.
There was just an article in the journal PNAS [ http://www.pnas.org/content/107/7/2763.abstract ] about how new methods further characterized the compounds in Murchison. Up to now, we knew of hundreds in it. Yet, because of new techniques, they’ve found tens of thousands. And that made me think that yes, we ought to redo the spark discharge experiment. I suspect we’d also find tens of thousands of molecules that were in the prebiotic soup. That, hopefully, is the next project we’ll be pursuing.
© 2011 The New York Times Company (emphasis in original)
http://www.nytimes.com/2010/05/18/science/18conv.html
===
The Pre-History of Life: elegantly simple organizing principles seen in ribosomes
Analysis of ribosome structures (shown on the left) from four different species revealed a non-random affinity between anticodon-containing RNA triplets and their respective amino acids (shown on the right).
Courtesy of David Johnson, Salk Institute for Biological Studies.
Ribosomes bring together all the mechanical machinery necessary for translation. They couple tRNAs to their proper codons on the mRNA and facilitate the formation of peptide bonds between amino acids.
Image: Courtesy of Jamie Simon, Salk Institute for Biological Studies
April 12, 2010
LA JOLLA, CA—With few exceptions, all known forms of life on our planet rely on the same genetic code to specify the amino acid composition of proteins. Although different hypotheses abound, just how individual amino acids were assigned to specific three-letter combinations or codons during the evolution of the genetic code is still subject to speculation.
Taking their hints from relics of this evolution left behind in modern cells, researchers at the Salk Institute for Biological Studies concluded that after only two waves of "matching" and some last minute fiddling, all 20 commonly used amino acids were firmly linked with their respective codons, setting the stage for the emergence of proteins with unique, defined sequences and properties.
Their findings, which will be published in next week's online edition of the Proceedings of the National Academy of Sciences [actually published the same day, abstract http://www.pnas.org/content/107/18/8298.abstract?sid=06798e39-fe19-48c6-b37c-ea4f230b93ea , full text http://www.pnas.org/content/107/18/8298.full , full text pdf http://www.pnas.org/content/107/18/8298.full.pdf - http://www.pnas.org/content/107/18/8298.full.pdf+html ], provide the first in vivo data shedding light on the origin and evolution of the genetic code.
"Although different algorithms, or codes, were likely tested during a long period of chemical evolution, the modern code proved so robust that, once it was established, it gave birth to the entire tree of life," says the study's lead author Lei Wang [ http://www.salk.edu/faculty/wang.html ], Ph.D., an assistant professor in the Chemical Biology and Proteomics Laboratory.
"But the universality of the code makes it very hard for researchers to study its formation since there are no organisms using a primitive or intermediate genetic code that we could analyze for comparison," he explains.
Cells provide a dazzling variety of functions that cover all of our body's needs, yet they make do with a very limited number of molecular building blocks. With few exceptions, all known forms of life use the same common 20 amino acids—and only those 20—to keep alive organisms as diverse as humans, earthworms, tiny daisies, and giant sequoias.
Each of the 20 amino acids is matched to its own carrier molecule known as transfer RNA (tRNA). During protein synthesis, which is coordinated by so-called ribosomes, amino acids are brought out one by one by their respective tRNAs and inserted in the growing protein chain according to the instructions spelled out in the universal language of life—the genetic code. The code is "read" with the help of anticodons embedded in each tRNA, which pair up with their codon-counterparts.
Several hypotheses have been put forward to explain why codons are selectively assigned to specific amino acids. "One of the theories, the stereochemical hypothesis, gained some traction when researchers could show that short codon- or anticodon-containing polynucleotide molecules like to interact with their respective amino acids," says graduate student and first author David B. F. Johnson.
If chemical or physical interactions between amino acids and nucleotide indeed drove the formation of the genetic code, Johnson reasoned, then he should be able to find relics of this mutual affinity in modern cells. He zoomed in on ribosomes, large complexes consisting of some 50 proteins interacting closely with ribosomal RNAs.
"Also, the ribosome emerged from an early evolutionary stage of life to help with the translation of the genetic code before the last universal common ancestor," explains Wang, "and therefore is more likely to serve as a molecular fossil that preserved biological evidence."
When Wang and Johnson probed bacterial ribosomes for imprints of the genetic code, they found evidence that direct interactions between amino acids and nucleotide triplet anticodons helped establish matching pairs. "We now believe that the genetic code was established in two different stages," says Johnson.
Their data does not shed much light on the early code, consisting of prebiotically available amino acids—the kind generated in Stanley Miller's famous "zap"-experiment. But once some primitive translational mechanism had been established, new amino acids were added to the mix and started infiltrating the genetic code based on specific amino acid/anticodon interactions.
"We found evidence that a few amino acids were reassigned to a different codon but once the code was in place it took over," says Johnson. "It might not have been the best possible solution but the only one that was viable at the time."
The work was supported in part by the Searle Scholar Program, the Beckman Young Investigator Program and the National Institutes of Health Director's New Innovator Award.
*
About the Salk Institute for Biological Studies
The Salk Institute for Biological Studies is one of the world's preeminent basic research institutions, where internationally renowned faculty probe fundamental life science questions in a unique, collaborative, and creative environment. Focused both on discovery and on mentoring future generations of researchers, Salk scientists make groundbreaking contributions to our understanding of cancer, aging, Alzheimer's, diabetes and infectious diseases by studying neuroscience, genetics, cell and plant biology, and related disciplines.
Faculty achievements have been recognized with numerous honors, including Nobel Prizes and memberships in the National Academy of Sciences. Founded in 1960 by polio vaccine pioneer Jonas Salk, M.D., the Institute is an independent nonprofit organization and architectural landmark.
© 2011 Salk Institute for Biological Studies
http://www.salk.edu/news/pressrelease_details.php?press_id=417 [also at e.g. http://esciencenews.com/articles/2010/04/12/the.pre.history.life.elegantly.simple.organizing.principles.seen.ribosomes ]
===
A Simple Solution to Life's Origin
The natural enantiomer of the RNA precursor molecules formed a crystal structure visible to the naked eye.
Credit: UC Merced
Model of an RNA molecule.
Image Credit: Lawrence Berkeley National Laboratory
Summary: A new study shows how a relatively simple combination of naturally occurring sugars and amino acids offers a plausible route to the building blocks of life.
Origin & Evolution of Life
Posted: 08/12/11
Source: UC Merced [ http://www.ucmerced.edu/ ] press release [ http://www.ucmerced.edu/news/study-builds-plausible-scenario-origin-life-earth ]
A relatively simple combination of naturally occurring sugars and amino acids offers a plausible route to the building blocks [ http://astrobiology.nasa.gov/roadmap ] of life, according to a paper published in Nature Chemistry co-authored by a professor at the University of California, Merced.
The study [ http://www.nature.com/nchem/journal/v3/n9/full/nchem.1108.html ], "A Route to Enantiopure RNA Precursors from Nearly Racemic Starting Materials," shows how the precursors to RNA could have formed on Earth before any life existed. It was authored by Jason E. Hein, Eric Tse and Donna G. Blackmond, a team of researchers with the Scripps Research Institute. Hein is now a chemistry professor with UC Merced. The paper was published online Sunday.
Biological molecules, such as RNA and proteins, can exist in either a natural or unnatural form, called enantiomers. By studying the chemical reactions carefully, the research team found that it was possible to generate only the natural form of the necessary RNA precursors by including simple amino acids.
"These amino acids changed how the reactions work and allowed only the naturally occurring RNA precursors to be generated in a stable form," said Hein. "In the end, we showed that an amazingly simple result emerged from some very complex and interconnected chemistry."
The natural enantiomer of the RNA precursor [ http://www.astrobio.net/exclusive/137/unfamiliar-life ] molecules formed a crystal structure visible to the naked eye. The crystals are stable and avoid normal chemical breakdown. They can exist until the conditions are right for them to change into RNA.
The study was led by Blackmond and builds on the work of John D. Sutherland and Matthew W. Powner published in 2009 and covered by outlets such as The New York Times and Wired. Sutherland is a chemist with Cambridge's Medical Research Council Laboratory of Molecular Biology. Powner is a post-doctoral scholar with Harvard University.
Copyright © 2011, Astrobio.net
http://www.astrobio.net/pressrelease/4148/a-simple-solution-to-lifes-origin
===
Hunting The Replicators: How Did Life Begin?
How did this get started?
AJC1 / http://flic.kr/p/XM1U9
by Adam Frank
August 16, 2011 04:25 pm
Amoebas are alive but rocks are not. What is the difference?
For many people, one of the greatest difficulties in understanding the scientific narrative of cosmic evolution is how nature took the path from non-life to life. This question of "abiotic genesis" haunts many a science and religion debate with advocates of scriptural literalism unwilling to grant that natural processes, unmediated by a higher intelligence, could have taken "dead" matter and created living material.
Now the path from "just" molecules to "something more" has gotten a bit more clear as researchers take a crucial step toward building the holy of holies: a self-replicating molecule.
Part of this story, however, is the funny thing that happened to scientists studying the origin of life over the last 80 years. Their perspectives were profoundly rewired.
In the 1950s, chemists Stanley Miller and Harold Urey performed a brilliant and brilliantly illustrative experiment [ http://www.chem.duke.edu/~jds/cruise_chem/Exobiology/miller.html ]. Miller and Urey created a simulated version of the early Earth in a test tube. An "atmosphere" of hydrogen, ammonia and methane was created in one flask. An "ocean" of liquid water was held in a separate flask. The two were connected and a high voltage discharge was set up in the atmospheric flask to act as lightning (and a source of UV light).
After running the experiment for a week, Miller and Urey found a brown goo had formed in their test-tube ocean. When they analyzed the goo they found it formed a rich soup of pre-biotic molecules. Amino acids, sugars and lipids (the basis of fat) had all been generated. The Miller-Urey experiment was hailed as instant classic of biochemistry and proof that, in principle, non-living material along with the natural processes of physics and chemistry could create the molecular basis of life. All that had to be added, scientists argued, was time and then life would have its start.
With time, however, scientists realized that there had to be more to the story of abiotic genesis. While the Miller-Urey experiment was revolutionary, as researchers came to understand the links between the molecular engines of life —DNA, RNA and proteins — it was clear their perspective had to shift. As the astrobiologist [ http://en.wikipedia.org/wiki/Astrobiology ] Chris McKay likes to put it, scientists eventually saw the problem was not just about chemistry but also about information processing as well.
As everyone knows, DNA constitutes the molecular blueprint for life. With the help of the molecule RNA, DNA can be read and transformed into proteins that are the workhorses of life. Proteins can fold up into a remarkable array of shapes giving them the ability to do everything from providing stable cellular structures to catalyzing chemical reactions when, and only when, they are needed.
Obviously, getting life started means getting a self-replicating molecule. That molecule must be capable of storing information about its own structure, operations and, of course, replication. DNA might seem the obvious choice, but it cannot replicate alone. DNA needs RNA and proteins to make copies of itself. That machinery could not have existed on the early Earth. Thus researchers had to find a molecule that could have formed on its own and with its own software.
As far back as 30 years ago RNA, a simpler molecule than DNA, was suggested as a candidate. Once it was recognized that RNA could fold up (like proteins) in ways that led to self-reactions, researchers saw the potential for an "RNA World [ http://www.panspermia.org/rnaworld.htm ]". The idea was that the early Earth must have been awash in RNA molecules building copies of themselves and enhancing their own abilities to store, process and act on information.
A key bottleneck in the process was finding examples of RNA with the "software" to process large sets of instructions and fully replicate themselves. This step is crucial because, for example, if you have a robot that can only build a hundredth of a robot then the dreaded robot apocalypse is probably still a long way away. Likewise, getting that first truly self-replicating molecule is the first true step towards evolution. Scientists have, up till recently, had a hard time finding an example of RNA that had the self-replicating chops.
But, as New Scientist [ http://www.newscientist.com/article/mg21128251.300-first-life-the-search-for-the-first-replicator.html ( http://www.newscientist.com/article/mg21128251.300-first-life-the-search-for-the-first-replicator.html?full=true ) (next below)] reports, researcher Philipp Holliger in Cambridge England has now taken a key step forward. Together with his collaborators, Holliger has created an RNA enzyme called tC19Z that can make copies of molecules almost half its own own length. If this does not sound impressive you need to understand that tC19Z is factor of 5 more capable than previous attempts at self replicating RNA. It's close enough in its ability to make endless copies of itself to make the RNA world seem like nature's best choice for creating life.
While we will never have molecular fossils that can tell us exactly what did happen four billion years ago, our progress in molecular biology is now allowing us to see what likely could have happened. Most importantly these new results are part of the long paradigm shift recognizing that living systems are as much about information processing as they are about moving material around.
Long live the replicators — whatever and wherever they are.
Copyright 2011 NPR (emphasis in original)
http://www.npr.org/blogs/13.7/2011/08/16/139673287/hunting-the-replicators-how-did-life-begin [with comments]
===
First life: The search for the first replicator
Dawn of the living
(Image: Gina and Matt)
Life must have begun with a simple molecule that could reproduce itself – and now we think we know how to make one
by Michael Marshall
15 August 2011
4 BILLION years before present: the surface of a newly formed planet around a medium-sized star is beginning to cool down. It's a violent place, bombarded by meteorites and riven by volcanic eruptions, with an atmosphere full of toxic gases. But almost as soon as water begins to form pools and oceans on its surface, something extraordinary happens. A molecule, or perhaps a set of molecules, capable of replicating itself arises.
This was the dawn of evolution. Once the first self-replicating entities appeared, natural selection kicked in, favouring any offspring with variations that made them better at replicating themselves. Soon the first simple cells appeared. The rest is prehistory.
Billions of years later, some of the descendants of those first cells evolved into organisms intelligent enough to wonder what their very earliest ancestor was like. What molecule started it all?
As far back as the 1960s, a few of those intelligent organisms began to suspect that the first self-replicating molecules were made of RNA, a close cousin of DNA. This idea has always had a huge problem, though - there was no known way by which RNA molecules could have formed on the primordial Earth. And if RNA molecules couldn't form spontaneously, how could self-replicating RNA molecules arise? Did some other replicator come first? If so, what was it? The answer is finally beginning to emerge.
When biologists first started to ponder how life arose, the question seemed baffling. In all organisms alive today, the hard work is done by proteins. Proteins can twist and fold into a wild diversity of shapes, so they can do just about anything, including acting as enzymes, substances that catalyse a huge range of chemical reactions. However, the information needed to make proteins is stored in DNA molecules. You can't make new proteins [ http://www.newscientist.com/article/mg15220578.100-cellular-factories.html ] without DNA, and you can't make new DNA without proteins. So which came first, proteins or DNA?
The discovery in the 1960s that RNA could fold like a protein, albeit not into such complex structures, suggested an answer. If RNA could catalyse reactions as well as storing information, some RNA molecules might be capable of making more RNA molecules. And if that was the case, RNA replicators would have had no need for proteins. They could do everything themselves.
It was an appealing idea, but at the time it was complete speculation. No one had shown that RNA could catalyse reactions like protein enzymes. It was not until 1982, after decades of searching, that an RNA enzyme was finally discovered. Thomas Cech of the University of Colorado in Boulder found [ http://www.newscientist.com/article/mg12416871.900-prizewinners-came-by-separate-paths-to-understand-rna.html ] it in Tetrahymena thermophila [ http://www.newscientist.com/article/dn20191-zoologger-the-hairy-beast-with-seven-fuzzy-sexes.html ], a bizarre single-celled animal with seven sexes (Science, vol 231, p 4737 [ http://www.sciencemag.org/content/231/4737/470 ]).
After that the floodgates opened. People discovered ever more RNA enzymes in living organisms and created new ones in their labs. RNA might be not be as good for storing information as DNA, being less stable, nor as versatile as proteins, but it was turning out to be a molecular jack of all trades. This was a huge boost to the idea that the first life consisted of RNA molecules that catalysed the production of more RNA molecules - "the RNA world", as Harvard chemist Walter Gilbert dubbed it 25 years ago (Nature, vol 319, p 618 [ http://www.nature.com/nature/journal/v319/n6055/abs/319618a0.html ]).
These RNA replicators may even have had sex. The RNA enzyme Cech discovered did not just catalyse any old reaction. It was a short section of RNA that could cut itself out of a longer chain [ http://www.newscientist.com/article/mg13217944.500-the-first-gene-on-earth.html ]. Reversing the reaction would add RNA to chains, meaning RNA replicators might have been able to swap bits with other RNA molecules. This ability would greatly accelerate evolution, because innovations made by separate lineages of replicators could be brought together in one lineage.
Evolving replicators
For many biologists the clincher came in 2000, when the structure of the protein-making factories [ http://www.newscientist.com/article/mg16722521.800-factory-of-life-gives-up-its-secrets.html ] in cells was worked out. This work confirmed that nestling at the heart of these factories is an RNA enzyme [ http://www.newscientist.com/article/dn17933-nobel-for-explaining-natures-protein-factories.html ] - and if proteins are made by RNA, surely RNA must have come first.
Still, some issues remained. For one thing, it remained unclear whether RNA really was capable of replicating itself. Nowadays, DNA and RNA need the help of many proteins to copy themselves. If there ever was a self-replicator, it has long since disappeared. So biochemists set out to make one, taking random RNAs and evolving them for many generations to see what they came up with.
By 2001, this process had yielded an RNA enzyme called R18 that could stick 14 nucleotides - the building blocks of RNA and DNA - onto an existing RNA, using another RNA as a template (Science, vol 292, p 1319 [ http://www.sciencemag.org/content/292/5520/1319 ]). Any self-replicating RNA, however, needs to build RNAs that are at least as long as itself - and R18 doesn't come close. It is 189 nucleotides long, but the longest RNA it can make contains just 20.
A big advance came earlier this year, when Philipp Holliger of the MRC Laboratory of Molecular Biology in Cambridge, UK, and colleagues unveiled an RNA enzyme called tC19Z [ http://www.newscientist.com/blogs/shortsharpscience/2011/04/rna-enzyme-makes-another-rna-e.html ]. It reliably copies RNA sequences up to 95 letters long, almost half as long as itself (Science, vol 332, p 209 [ http://www.sciencemag.org/content/332/6026/209 ]). To do this, tC19Z clamps onto the end of an RNA, attaches the correct nucleotide, then moves forward a step and adds another. "It still blows my mind that you can do something so complex with such a simple molecule," Holliger says.
So biologists are getting tantalisingly close to creating an RNA molecule, or perhaps a set of molecules, capable of replicating itself. That leaves another sticking point: where did the energy to drive this activity come from? There must have been some kind of metabolic process going on - but RNA does not look up to the job of running a full-blown metabolism.
"There's been a nagging issue of whether RNA can do all the chemistry," says Adrian Ferré-D'Amaré of the National Heart, Lung and Blood Institute in Bethesda, Maryland. RNA has only a few chemically active "functional groups", which limit it to catalysing just a few types of chemical reaction.
Functional groups are like tools - the more kinds you have, the more things you can do. Proteins have many more functional groups than RNAs. However, there is a way to make a single tool much more versatile: attach different bits to it, like those screwdrivers that come with interchangeable heads. The chemical equivalents are small helper molecules known as cofactors.
Proteins use cofactors to extend even further the range of reactions they can control. Without cofactors, life as we know it couldn't exist, Ferré-D'Amaré says. And it turns out that RNA enzymes can use cofactors too.
In 2003, Hiroaki Suga, now at the University of Tokyo, Japan, created an RNA enzyme that could oxidise alcohol, with help from a cofactor called NAD+ which is used by many protein enzymes (Nature Structural Biology, vol 10, p 713 [ http://www.nature.com/nsmb/journal/v10/n9/full/nsb964.html ]). Months later, Ronald Breaker of Yale University found that a natural RNA enzyme, called glmS, also uses a cofactor [ http://www.pnas.org/content/101/17/6421 ].
Many bacteria use glmS, says Ferré-D'Amaré, so either it is ancient or RNA enzymes that use cofactors evolve easily. Either way, it looks as if RNA molecules would have been capable of carrying out the range of the reactions needed to produce energy.
So the evidence that there was once an RNA world is growing ever more convincing. Only a few dissenters remain [ http://www.newscientist.com/article/mg15120373.900-let-there-be-life.html ]. "The naysayers about the RNA world have lost a lot of ground," says Donna Blackmond of the Scripps Research Institute in La Jolla, California. But there is still one huge and obvious problem: where did the RNA come from in the first place?
RNA molecules are strings of nucleotides, which in turn are made of a sugar with a base and a phosphate attached. In living cells, numerous enzymes are involved in producing nucleotides and joining them together, but of course the primordial planet had no such enzymes. There was clay, though. In 1996, biochemist Leslie Orgel showed that when "activated" nucleotides - those with an extra bit tacked on to the phosphate - were added to a kind of volcanic clay, RNA molecules up to 55 nucleotides long formed (Nature, vol 381, p 59 [ http://www.nature.com/nature/journal/v381/n6577/abs/381059a0.html ]). With ordinary nucleotides the formation of large RNA molecules would be energetically unfavourable, but the activated ones provide the energy needed to drive the reaction.
This suggests that if there were plenty of activated nucleotides on the early Earth, large RNA molecules would form spontaneously. What's more, experiments simulating conditions on the early Earth and on asteroids show that sugars, bases and phosphates [ http://www.newscientist.com/article/dn14966-volcanic-lightning-may-have-sparked-life-on-earth.html ] would arise naturally too. It's putting the nucleotides together that is the hard bit; there does not seem to be any way to join the components without specialised enzymes. Because of the shapes of the molecules, it is almost impossible for the sugar to join to a base, and even when it does happen, the combined molecule quickly breaks apart.
This apparently insurmountable difficulty led many biologists to suspect to RNA was not the first replicator after all. Many began exploring the possibility that the RNA world was preceded by a TNA world, or a PNA world, or perhaps an ANA world. These are all molecules similar to RNA but whose basic units are thought to have been much more likely to form spontaneously. The big problem with this idea is that if life did begin this way, no evidence of it remains. "You don't see a smoking gun," says Gerald Joyce, also of the Scripps Research Institute.
In the meantime John Sutherland, at the MRC Laboratory of Molecular Biology, has been doggedly trying to solve the nucleotide problem. He realised that researchers might have been going about it the wrong way. "In each nucleotide, you see a sugar, a base and a phosphate group," he says. "So you assume you need to make those building blocks first and then stick them together... and it doesn't work."
Instead he wondered whether simpler molecules might assemble into a nucleotide without ever becoming sugars or bases. In 2009, he proved it was possible. He took half a sugar and half a base, and stuck them together - forming the crucial sugar-base link that everyone had struggled with. Then he bolted on the rest of the sugar and base. Sutherland stuck on the phosphate last, though he found that it needed to be present in the mixture for the earlier reactions to work (Nature, vol 459, p 239 [ http://www.nature.com/nature/journal/v459/n7244/full/nature08013.html ]).
Goldilocks chemistry
Sutherland was being deliberately messy by including the phosphate from the start, but it gave the best results. That's encouraging: the primordial Earth was a messy place and it may have been ideal for making nucleotides. Sutherland now suspects there is a "Goldilocks chemistry" [ http://www.newscientist.com/article/mg20928023.900-primordial-pacman-oil-droplet-hints-at-lifes-origin.html ] - not too simple, not too complex - that would produce many key compounds from the same melting pot.
"Sutherland had a real breakthrough," Holliger says. "Everyone else was barking up the wrong tree."
The issue isn't entirely solved yet. RNA has four different nucleotides, and so far Sutherland has only produced two of them. However, he says he is "closing in" on the other two. If he succeeds, it will show that the spontaneous formation of an RNA replicator is not so improbable after all, and that the first replicator was most likely made of RNA.
Many questions remain, of course. Where did the first replicators arise? What was the first life like? How did the transition to DNA and proteins, and the development of the genetic code, occur? We may never know for sure but many promising avenues are being explored. Most biologists think there must have been something like a cell right from the start, to contain the replicator and keep its component parts together. That way, individuals could compete for resources and evolve in different ways.
Jack Szostak of Harvard University has shown that the same clay that produces RNA chains also encourages the formation of membrane-bound sacs [ http://www.newscientist.com/article/dn4307-clays-matchmaking-could-have-sparked-life.html ] rather like cells that enclose cells. He has grown "proto-cells" that can carry RNA and even divide [id.] without modern cellular machinery.
Another idea is that life began in alkaline hydrothermal vents on the sea floor [ http://www.newscientist.com/article/mg20427306.200-was-our-oldest-ancestor-a-protonpowered-rock.html ]. Not only are these vents laced with pores and bubbles, but they also provide the same kind of electrochemical gradient that drives energy production in cells to this day. Conditions may have been ideal for producing long RNA chains.
Holliger has a rather surprising idea: maybe it all happened in ice. At the time life began, the sun was 30 per cent dimmer than today. The planet would have frozen over if the atmosphere hadn't been full of greenhouse gases, and there may well have been ice towards the poles.
Cold RNA lasts longer, and ice has many other benefits. When water laced with RNA and other chemicals is cooled, some of it freezes while the rest becomes a concentrated brine running around the ice crystals. "You get little pockets within the ice," Holliger says. Interestingly, the R18 RNA enzyme works better in ice than at room temperature (Nature Communications, DOI: 10.1038/ncomms1076 [ http://www.nature.com/ncomms/journal/v1/n6/full/ncomms1076.html ]).
Right now, there's no way to choose between these options. No fossilised vestiges remain of the first replicators as far as we know. But we can try recreating the RNA world to demonstrate how it might have arisen. One day soon, Sutherland says, someone will fill a container with a mix of primordial chemicals, keep it under the right conditions, and watch life emerge. "That experiment will be done."
Michael Marshall is a reporter for New Scientist
© Copyright Reed Business Information Ltd.
http://www.newscientist.com/article/mg21128251.300-first-life-the-search-for-the-first-replicator.html [ http://www.newscientist.com/article/mg21128251.300-first-life-the-search-for-the-first-replicator.html?full=true ] [with comments]
===
‘It’s Alive! It’s Alive!’ Maybe Right Here on Earth
Video [embedded]
Life Out There: Eden in a Test Tube
To better recognize extraterrestrial life should they come upon it, scientists are working to create simple life forms in a lab. But, as Dennis Overbye reports, they first have to agree what life is.
Jim Wilson/The New York Times
Dr. Joyce in his lab at the Scripps Research Institute in San Diego.
Jim Wilson/The New York Times
By DENNIS OVERBYE
Published: July 27, 2011
SAN DIEGO — Here in a laboratory perched on the edge of the continent, researchers are trying to construct Life As We Don’t Know It in a thimbleful of liquid.
Generations of scientists, children and science fiction fans have grown up presuming that humanity’s first encounter with alien life will happen in a red sand dune on Mars, or in an enigmatic radio signal from some obscure star.
But it could soon happen right here on Earth, according to a handful of chemists and biologists who are using the tools of modern genetics to try to generate the Frankensteinian spark that will jump the gap separating the inanimate and the animate. The day is coming, they say, when chemicals in a test tube will come to life.
By some measures, Gerald F. Joyce, a professor at the Scripps Research Institute here, has already crossed that line, although he would be the first to say he has not — yet.
Biologists do not agree on what the definition of life should be or whether it is even useful to have one. But most do agree that the ability to evolve and adapt is fundamental to life. And they also agree that having a second example of life could provide insight to how it began and how special life is or is not in the universe, as well as a clue for how to recognize life if and when we do stumble upon it out there among the stars.
“Everything we know about life is based on studies of life on Earth,” said Chris McKay, a researcher at NASA’s Ames Research Laboratory in Mountain View, Calif.
Dr. Joyce said recently: “It drives me crazy when astronomers say, ‘Surely the universe is pregnant with life.’ If we have an Earthlike planet, what are the chances of life arising? Is it one in a million? Is it one in two? I don’t see how you can say.”
He continued, “If you had a second example of life, even if it were synthetic, you might know better. I’m betting we’re just going to make it.”
Four years ago Dr. Joyce and a graduate student, Tracey A. Lincoln, now a researcher at the University of Massachusetts Medical School, evolved a molecule in a test tube that could replicate and evolve all by itself, swapping little jerry-built genes in a test tube forever, as long as it was supplied with the right carefully engineered ingredients.
An article in the Joyce Laboratory newsletter called it “The Immortal Molecule.” Dr. Joyce’s molecule is a form of RNA, or ribonucleic acid, which plays Robin to DNA’s Batman in Life As We Do Know It, assembling proteins in accordance with the blueprint encoded in DNA. Neither RNA nor DNA is alive by itself, any more than any other chemical, like bleach, or a protein. But in Dr. Joyce’s test tube, his specially engineered RNA molecule comes close, copying itself over and over, and evolving.
But, Dr. Joyce says, “We really would hope for more from our molecules than just replicating.”
Reproduction is the job of any life, he explained, but Earthly organisms have evolved a spectacular set of tricks to improve the odds of success — everything from peacock feathers to whale songs. Dr. Joyce’s molecules have not yet surprised him by striking out on their own to invent the molecular equivalent of writing a hit pop song.
It is only a matter of time, he said, before they do.
“Our job is to give them the running room to do that,” Dr. Joyce said.
The deeper philosophical and intellectual ramifications of test tube life are as enormous as they are unknown. The achievement would probably not come with sci-fi drama, say scientists who are squeamish about such matters anyway, saying such speculation is beyond their pay grade. No microbe is going to leap out of the Petri dish and call home, or turn the graduate students into zombies. Indeed, given the human penchant for argument and scientists’ habit of understatement, it could be years before everybody agrees it has been done.
“The ability to synthesize life will be an event of profound importance, like the invention of agriculture or the invention of metallurgy,” Freeman Dyson, a mathematician and physicist at the Institute for Advanced Study in Princeton, wrote in an e-mail. “Nobody can tell in advance what will come of it.”
On Earth, all life as we know it is based on DNA, the carbon-based molecule that contains the instructions for making and operating living cells in a four-letter alphabet along its double-helix spine.
The possibilities of a second example of life are as deep as the imagination. It could be based on DNA that uses a different genetic code, with perhaps more or fewer than four letters; it could be based on some complex molecule other than DNA, or more than the 20 amino acids from which our own proteins are made, or even some kind of chemistry based on something other than carbon and the other elements that we take for granted, like phosphorous or iron. Others wonder whether chemistry is necessary at all. Could life manifest itself, for example, in the pattern of electrically charged dust grains in a giant interstellar cloud, as the British astronomer and author Fred Hoyle imagined in his novel “The Black Cloud”?
Dr. Joyce said that his RNA replicators would count as such a “second example, albeit one constructed as a homage to our ancient ancestors.”
So far, he said, his work with Dr. Lincoln has shown that manmade molecules can evolve over successive generations. “They can pass information from parent to progeny, they can mutate,” Dr. Joyce said. “They can win or die. The molecules are doing it all. We’re just keeping the lights on.”
Dr. Joyce’s molecules may not be clever enough yet to qualify as life in his view, but all sorts of alternatives are being explored in other labs.
Some researchers, like Steven Benner of the Foundation for Applied Molecular Evolution in Florida, are constructing and experimenting with forms of DNA that use coding alphabets of more than four letters. J. Craig Venter, who helped spearhead the decoding of the human genome and now works as president of the J. Craig Venter Institute, recently used store-bought chemicals to reconstruct the genome of a bacterial goat parasite and put it in another bacterium, where it took over, churning out copies of itself with Dr. Venter’s watermark inscribed in its gene code.
In a related vein, George Church and Farren Isaacs of the Harvard Medical School recently reported that they had reprogrammed the genome of an E. Coli bacterium [ http://www.nytimes.com/2011/07/15/health/15genome.htm ], opening up the possibility of incorporating new features into the ubiquitous little bug. Dr. Joyce called the work “really macho molecular biotechnology.”
Jack Szostak of Harvard Medical School and his collaborators have embarked on an ambitious project to build an artificial cell that can replicate and presumably evolve. Dr. Benner wrote in an e-mail, “In my view, a terran laboratory will make synthetic life before NASA or the E.S.A. finds it elsewhere,” referring to the European Space Agency. He added, “And a lot before, given the disassembling of NASA by the current administration.”
According to modern science, life on Earth originated about 3.8 billion years ago, perhaps in a warm pond, as Darwin speculated, or perhaps in a boiling, bubbling mud bath or a scorching volcanic vent way under the sea. The first inhabitant of this Eden, chemists suspect, was RNA.
In today’s world RNA runs errands for DNA. Like DNA, RNA encodes genetic information. Unlike DNA, however, RNA can also catalyze chemical reactions between other molecules, chopping them up or binding them together, a task mostly performed by proteins in modern organisms.
In 1962, the M.I.T. biologist Alexander Rich suggested that RNA could have played both roles — blueprint and machinery — at the beginning. Scientists cannot prove that this is how life arose on Earth, but they can do the next best thing. They can make their own RNA and see if they can then breathe life into it.
Enter Dr. Joyce, who says he came to his vocation by reading “Gravity’s Rainbow,” Thomas Pynchon’s 1973 novel about rockets and death in World War II, while he was a student at the University of Chicago. The last section of that book, he pointed out, is called “The Counterforce,” about pockets of life and love carving order out of the rubble of wartime Europe. For biologists the counterforce creating order and life out of chaos is simply Darwinian evolution, Dr. Joyce explained. “I wanted to be a member of the counterforce.”
At the center of the Joyce lab experiments is a T-shaped piece of RNA that has the ability to glue together other molecules of RNA. In 2002, Dr. Joyce and a postdoctoral fellow, Natasha Paul, configured it to recognize and glue together a pair of smaller molecules, essentially an L and a straight piece. When joined, those molecules would form a new copy of the original T-shaped molecule. It worked; the RNA was able to manufacture new versions of itself, but not fast enough to keep up with the original RNA’s natural tendency to fall apart. Essentially it was dying faster than it was reproducing. Dr. Joyce and Dr. Lincoln found a way to speed the process up, by having two complementary versions of the RNA manufacture each other.
“There was a day that it all happened,” said Dr. Joyce, namely Oct. 1, 2007, when as he puts it, the replicators “went critical,” and their population began growing exponentially.
The game, as he likes to say, was on. And it has never stopped. Dr. Joyce and his colleagues next proceeded to engineer a sort of March Madness for molecules. They synthesized 12 versions of the replicators, which could mutate and evolve to improve their ability to reproduce. The experimenters threw these into the pot, along with the appropriate “food” segments, to compete. “They just go at it,” Dr. Joyce explained.
By the end, the winning molecules were doubling their numbers every 15 minutes. Mistaken swaps had produced combinations, mutations, that had not been in the mix at the start. Most of the original versions almost completely disappeared. In short, the molecule evolved.
“Evolution is not a theory for us chemists,” Dr. Joyce said. “It’s what molecules do when they have the property to replicate and transmit information from parents to progeny.”
In a separate experiment the molecules were redesigned so that they would replicate only when another chemical was present. “That’s the app that’s going to pay for this,” said Dr. Joyce, explaining that the replicating molecules could be fashioned into sensors to detect pollutants or dangerous toxins in the environment. Dr. Joyce and his collaborators are now starting to run the same tournament with 256 versions of the replication enzyme. “We are pipetting madly,” he reported recently.
That means that there will be about 65,000 possible gene combinations that can emerge and try out their wings, which means things are getting interesting. As Dr. Lincoln said, “We’re knocking on the door, but we’re not quite there yet.” Sidney Altman [ http://www.yale.edu/mcdb/facultystaff/altman.html ], a Yale professor who shared a Nobel prize for discovering some of the talents of RNA, said that true test tube life could still be years away. “Gerry Joyce’s replicators are very clever molecules,” he said, but added that they were not self-sufficient enough to be alive.
Dr. Joyce said his team was working on having the replicating molecule invent a new ability, but he would not say what it was. Asked for an example of the kind of things he could teach his RNA to do, Dr. Joyce suggested it could take part in creating one of the ingredients for its own replication by adding together a pair of smaller molecules. “What would be cool,” he said, “would be if they could make their own food.” The key to more ability, he said, is complexity. His molecule has only two genes, compared with 25,000 in human beings, and experiments involved fiddling with four letters of these genes. The human genome has three billion letters.
“We have a little toy genome where we can have the complete book of life,” he said, “but the sentences only have two words.”
Dr. Joyce’s molecules will never catch up to the biosphere. But someday their genome may surprise their creator with a word — a trick or a new move in the game of almost life — that he has not anticipated. “If it would happen, it would do it for me, I would be happy,” Dr. Joyce said, adding, “I won’t say it out loud, but it’s alive.”
*
Life Out There
From Inanimate to Animate
Articles in this series examine the search for new forms of life in the universe.
http://topics.nytimes.com/top/news/science/series/life_out_there/index.html
*
© 2011 The New York Times Company
http://www.nytimes.com/2011/07/28/science/28life.html [ http://www.nytimes.com/2011/07/28/science/28life.html?pagewanted=all ] [comments at http://community.nytimes.com/comments/www.nytimes.com/2011/07/28/science/28life.html ]
===
Amino Acid Alphabet Soup
The amino acid sequence of a protein determines the higher levels of structure of the molecule. A single change in the amino acid sequence can have profound biological effects on the overall structure and function of the protein.
Image Credit: IACR
Amino acids have been found in meteorites, such as the Murchison meteorite.
Image Credit: NASA
How did life end up selecting which amino acids in the primordial soup worked best?
Image Credit: Peter Sawyer / Smithsonian Institution
[image from http://www.space.com/12665-alien-life-chemical-code-amino-acids.html ]
Comet Wild 2 imaged just after flyby. Samples of the gas and dust coming off of the comet collected by the Stardust spacecraft contained the amino acid glycine.
Credit: NASA/JPL
Astronomers have detected hydrogen cyanide and methanimine, two carbon compounds that can combine with water to make the amino acid glycine, in the Arp 220 galaxy.
Image Credit: NASA, ESA, and C. Wilson (McMaster University, Hamilton, Ontario, Canada)
[image from http://www.space.com/12665-alien-life-chemical-code-amino-acids.html ]
Summary: All life on Earth relies on a standard set of 20 amino acids to build the proteins that carry out life's essential actions. But did it have to be this way?
Origin & Evolution of Life
Posted: 08/18/11
Author: Clara Moskowitz
All life on Earth relies on a standard set of 20 molecules called amino acids to build the proteins that carry out life's essential actions. But did it have to be this way?
All living creatures on this planet use the same 20 amino acids, even though there are hundreds available in nature. Scientists therefore have wondered if life could have arisen based on a different set of amino acids. And what's more, could life [ http://astrobiology.nasa.gov/roadmap ] exist elsewhere that utilizes an alternate collection of building blocks?
"Life has been using a standard set of 20 amino acids to build proteins for more than 3 billion years," said Stephen J. Freeland of the NASA Astrobiology Institute at the University of Hawaii. "It's becoming increasingly clear that many other amino acids were plausible candidates, and although there's been speculation and even assumptions about what life was doing, there's been very little in the way of testable hypotheses."
So Freeland and his University of Hawaii colleague Gayle K. Philip devised a test to try to learn if the 20 amino acids Earth's life uses were randomly chosen, or if they were the only possible ones that could have done the job. Amino acids are molecules built primarily from carbon, hydrogen, oxygen, and nitrogen. They assemble in particular shapes and patterns to form larger molecules called proteins that carry out biological functions.
"Technically there is an infinite variety of amino acids," Freeland told Astrobiology Magazine. "Within that infinity there are lots more than the 20 that were available [when life originated on Earth] as far as we can tell."
Testing the possibilities
The researchers defined a likely pool of candidate amino acids from which life drew its 20. They started with the amino acids that have been discovered within the so-called Murchison meteorite, a space rock that fell in Murchison, Victoria in Australia in September 1969. The rock is thought to date from the early solar system, and to represent a sample of which compounds existed in the solar system and on Earth before life began.
The scientists then used computers to estimate the fundamental properties of the 20 amino acids life uses, such as size, charge and hydrophilicity, or the extent to which the molecules are attracted to water.
"We know that these three are important to the ways they build proteins," Freeland said.
Freeland and Philip analyzed whether these properties could have been achieved with as much coverage and efficiency with other combinations of 20 amino acids.
The researchers discovered that life seemingly did not choose its 20 building blocks randomly. "We found that chance alone would be extremely unlikely to pick a set of amino acids that outperforms life's choice," Freeland said.
Natural selection
In fact, the researchers think early life on Earth probably used a version of natural selection to choose these amino acids. Some combinations of other amino acids were likely tried, but none proved quite as fit, so no other combinations ended up producing the numbers of successful offspring that the existing set achieved.
"Here we found a very simple test that begins to show us that life knew exactly what it was doing," Freeland said. "This is consistent with the idea that there was natural selection going on."
Getting at the question of why nature chose the 20 amino acids it did is experimentally difficult, said Aaron Burton, a NASA Postdoctoral Program Fellow who works as an astrochemist at NASA's Goddard Space Flight Center in Greenbelt, Md.
"Although a number of experiments [ http://www.astrobio.net/pressrelease/979/expanding-the-genetic-code ] have shown that unnatural amino acids can be incorporated into the genetic alphabet of organisms, it may never be possible to experimentally simulate sufficient evolutionary time periods to truly compare alternate amino acid alphabets," said Burton, who was not involved in the new study. "As a result, studies such as those presented by Philip and Freeland offer interesting insights and provide a framework for formulating hypotheses that can actually be tested in the lab."
Amino acids in meteorites
Right now the race is on to directly find amino acids elsewhere in the solar system. Some hints that they abound have been found on meteorites that have landed on Earth from outer space, as well as from missions such as NASA's Stardust probe, which sampled the coma [ http://www.astrobio.net/pressrelease/3220/glycine-in-the-grid ] of comet Wild 2 in 2004.
"All signs are that amino acids are going to be found throughout the galaxy," Freeland said. "They are apparently obvious building blocks with which to construct life. What we're finding hints at a certain level of predictability in the way things turned out."
The question of life's amino acid toolbox is interesting not just in trying to trace the origin of the life on Earth, but in wondering whether life exists on other planets, and if so, what form it takes. Scientists are particularly curious about how a different set of amino acid building blocks would result in different characteristics in the life it creates.
"That is the biggest question of all," Freeland said. "We're trying to find a way to ask, if you change the set of amino acids with which we're building, what effect does that have on the proteins you can build. The most interesting thing is, nobody knows."
Philip and Freeland reported their findings in a paper published [ http://www.liebertonline.com/doi/abs/10.1089/ast.2010.0567 ] in the April 19 issue of the journal Astrobiology.
Copyright © 2011, Astrobio.net
http://www.astrobio.net/exclusive/4161/amino-acid-alphabet-soup
===
How Simple Life Got Complicated?
Studying yeast cells has provided many scientific advancments in biology over the years. Yeast has even made it into space. Above are scanning electron micrographs of Saccharomyces cerevisiae (yeast) grown in microgravity (A) and gravity (B).
Credit: NASA/JSC
Yeast is most famous for its fermentative abilities, which are essential in the production of wine and many other alcoholic beverages. Now, the microorganism is helping scientists understand the development of multicellular life.
Summary: New research shows how single-celled organisms may have initially banded together into multicellular groups.
Origin & Evolution of Life
Posted: 08/13/11
Source: Public Library of Science press release [ http://www.eurekalert.org/pub_releases/2011-08/plos-ith080311.php ]
A new study has created an analog of what researchers think the first multicellular cooperation might have looked like, showing that yeast [ http://www.astrobio.net/interview/367/hitch-hikers-guide-to-biologys-second-datum ] cells—in an environment that requires them to work for their food—grow and reproduce better in multicellular clumps than singly.
A team of researchers, led by Harvard professor Andrew Murray, found that cells of brewer's yeast [ http://www.astrobio.net/pressrelease/3970/a-prehistoric-microbial-world-in-a-glass-of-wine ] that clumped together were able more effectively to manipulate and absorb sugars in their environment than were similar cells that lived singly. The experiments showed that in environments where the yeast's sugar food source is dilute and the number of cells is small, the ability to clump together allowed cells that otherwise would have remained hungry and static to grow and divide.
The work, published August 9 [ http://www.plosbiology.org/article/info%3Adoi%2F10.1371%2Fjournal.pbio.1001122 ] in the online, open access journal PLoS Biology, used the yeast Saccharomyces cerevisiae [ http://www.astrobio.net/pressrelease/979/expanding-the-genetic-code ], which is commonly used in brewing and bread-making and has long been used by scientists as a model organism for understanding single-celled life. Murray and colleagues devised a series of experiments that presented two problems for the yeast cells to solve if they were to take in enough food to grow and divide: the first was how to change their food from an unusable form to a usable form; the second was how to actually take in this food.
The researchers put the yeast in a solution of sucrose—plain old table sugar—which is composed of two simpler sugars, glucose and fructose. Yeast lives on sugar, but the sucrose can't get through the membrane that surrounds the cell. So the yeast makes an enzyme called invertase to chop the sucrose into glucose and fructose, each of which can enter the cell using gate-keeping molecules, called transporters, that form part of the membrane.
The second problem was how to get the glucose and fructose from the place where they were split apart by invertase to the transporters in the cell membrane. The only way to bridge the gap is through diffusion, an inefficient process. Researchers calculated that once a cell makes invertase and chops the larger sugar down to usable bits, only one sugar molecule in 100 would be captured by the cell that made it.
They also calculated that, working alone, a single yeast cell in a dilute solution of sucrose would never take in enough glucose and fructose to be able to grow and divide. But by cooperating, clumps of yeast in that same solution might have a chance. With several cells in proximity, all releasing invertase to create smaller sugars, these cooperating yeast cells [ http://www.astrobio.net/pressrelease/2598/making-artificial-bacteria ] would increase the density of those sugars near the clump, increasing the chances that each cell could take in enough to grow and divide. Sure enough, when the researchers tested these hypotheses on two strains of yeast, they found that the strain which clumped cells together was growing and dividing, while the yeast cells living alone were not.
Murray said the work offers one explanation as to why single-celled organisms might have initially banded together deep in the history of life [ http://astrobiology.nasa.gov/roadmap ], although it's impossible to prove conclusively that this is what happened. "Because there is an advantage to sticking together under these circumstances, and because we know that lots of single-celled organisms make enzymes to liberate goods from their environment, this may be the evolutionary force that led to multicellularity," Murray said. Although, he continued, "short of inventing time travel and going back several billion years to see if this is how it happened…this is going to remain speculation."
Copyright © 2011, Astrobio.net
http://www.astrobio.net/pressrelease/4150/how-simple-life-got-complicated
===
(linked in):
http://investorshub.advfn.com/boards/read_msg.aspx?message_id=64150018 and preceding and following
from earlier/elsewhere this string, http://investorshub.advfn.com/boards/read_msg.aspx?message_id=66812099 and preceding and following, and http://investorshub.advfn.com/boards/read_msg.aspx?message_id=66795926 and preceding and following
http://investorshub.advfn.com/boards/read_msg.aspx?message_id=44715115 and preceding (and any future following)
http://investorshub.advfn.com/boards/read_msg.aspx?message_id=66175494 (and preceding) and following (in particular http://investorshub.advfn.com/boards/read_msg.aspx?message_id=66343804 )
http://investorshub.advfn.com/boards/read_msg.aspx?message_id=66819316 and preceding (and any future following)

One of the earliest known forms of life on Earth is stromatolites, which consist of cyanobacteria (blue-green algae). Fossil records of stromatolites date back 3.5 billion years. This photo shows modern stromatolites in Shark Bay, Western Australia.
Image credit: Paul Harrison.
By Lisa Zyga
December 9, 2008
(PhysOrg.com) -- Today, scientists understand pretty well how life evolves, by mechanisms based on Darwin’s theory of natural selection for survival of the fittest. However, Darwin’s 1859 classic, On the Origin of Species, somewhat ironically doesn’t answer that very question – how species actually originated. And to this day, how that first tiny pool of chemicals twitched to life remains a puzzle.
In a recent study called “Why did life emerge?”, two scientists, son and father Arto Annila of the University of Helsinki and Erkki Annila of the Finnish Forest Research Institute, offer some insight into the general driving force of life’s origins in terms of thermodynamics. As they explain, all organisms are composed of molecules that assemble together via numerous chemical reactions. Just as heat flows from hot to cold, these molecules obey the universal tendency to diminish energy differences, so that the most likely chemical reactions are those in which energy flows “downhill” toward a stationary state, or chemical equilibrium.
Although the researchers don’t speculate on the specific chemical reactions that created life, they explain that the molecules involved most likely underwent a series of more and more complex reactions to minimize mutual energy differences between matter on Earth and with respect to high-energy radiation from Sun. The process eventually advanced so far that it cumulated into such sophisticated functional structures that could be called living.
“The most important idea in our study is that there is no distinction between animate and inanimate,” Arto Annila told PhysOrg.com. “Processes of life are, in their principles, no different from any other natural processes.”
In their study, which is published in the International Journal of Astrobiology, the researchers considered a primordial pool that contained some basic compounds. By reacting with one another and coupling with an external energy source such as the Sun, the compounds formed a chemical system. The compounds continually engaged in chemical reactions, thriving the most when capturing and distributing more and more of the Sun’s energy in the quest for a steady state. The evolutionary process was and still is non-deterministic, even chaotic, since the energy flows create energy differences that in turn affect the flows.
Due to random variations stemming from the chemical reactions, some novel compounds may have emerged in the primordial system. Some of these compounds (such as those involving carbon) might have been exceptionally good at creating energy flow, enabling the system to diminish energy differences very efficiently and reach a higher level of entropy. Compounds with these advantages would have gained ground during this period of primitive chemical evolution. But the scientists emphasize that identifying which exact compounds were key players during this period would be very difficult to determine.
“Today we may have only very little evidence left from the courses in the very distant past to deduce which chemical species went extinct, while others, more viable in energy transduction, emerged,” Arto Annila explained. In other words, this study focuses on why life emerged, not how.
What is more relevant, the scientists note, is the fact that the physical tendency to diminish energy differences makes no distinction between systems that are inanimate or animate. As the researchers explain, the order and complexity that characterize modern biological systems have no value in and of themselves, but structure and hierarchical organization emerged and developed because they provided paths for increasing energy flows.
The scientists give several examples of mechanisms associated with life that increase entropy. For instance, when systems (e.g. molecules) become entities of larger systems (e.g. cells) that participate in larger ranges of interactions to consume more free energy, entropy increases. Genetic code might have served as another primordial mechanism, acting as a catalyst that could increase energy flow toward greater entropy. Today, complex organisms have cellular metabolism, which is another mechanism that increases entropy, as it disperses energy throughout the organism and into the environment. The food chain in an ecosystem is another example of a mechanism for transferring energy on a larger scale.
In this sense, life is a very natural thing, which emerged simply to satisfy basic physical laws. Our “purpose,” so to speak, is to redistribute energy on the Earth, which is in between a huge potential energy difference caused by the hot Sun and cold space. Organisms evolve via natural selection, but at the most basic level, natural selection is driven by the same thermodynamic principle: increasing entropy and decreasing energy differences. The natural processes from which life emerged, then, are the same processes that keep life going – and they operate on all timescales.
“According to thermodynamics, there was no striking moment or no single specific locus for life to originate, but the natural process has been advancing by a long sequence of steps via numerous mechanisms so far reaching a specific meaning – life,” the researchers explained.
And because thermodynamics recognizes no specific moment, particular place, compound or reaction that would distinguish animate from inanimate, a search for ‘the birth of life’ seems like an ill-posed project, Arto Annila explained.
“Indeed, the quest for the origin of life seems a futile endeavor because life in its entirety is a natural process that has, according to the second law of thermodynamics, no definite beginning,” he said. “To ask how life started would be the same as to ask when and where did the first wind blow that quivered the surface of a warm pond.”
More information: Annila, Arto and Annila, Erkki. “Why did life emerge?” International Journal of Astrobiology 7 (3 & 4): 293-300 (2008) [abstract http://journals.cambridge.org/action/displayAbstract?fromPage=online&aid=2820924 and http://arxiv.org/abs/0910.2621 , full text pdf http://www.helsinki.fi/~aannila/arto/whylife.pdf and http://arxiv.org/ftp/arxiv/papers/0910/0910.2621.pdf ].
Copyright 2008 PhysOrg.com.
http://www.physorg.com/news148050302.html [with comments]
===
A Marine Chemist Studies How Life Began

Jeffrey L. Bada, 67, is the distinguished professor of marine chemistry at the University of California, San Diego. He studies how life began.
Robert Benson for The New York Times
A Conversation With Jeffrey L. Bada
By CLAUDIA DREIFUS
Published: May 17, 2010
Jeffrey L. Bada, 67, is the distinguished professor of marine chemistry at the University of California, San Diego. He studies how life began. We spoke for an hour during the American Association for the Advancement of Science’s annual meeting in San Diego last winter and again this month by telephone. An edited version of the two conversations follows:
Q. HAVE YOU ALWAYS BEEN INTERESTED IN THE CHEMISTRY OF LIFE?
A. No. When I started in graduate school in 1965, I had ideas about becoming a theoretical chemist, applying quantum mechanics to chemistry. But when I arrived at U.C.S.D., I met Stanley Miller, who’d been a student of Nobel Prize-winning chemist Harold Urey. In 1953, they completed the classic experiment on the chemical origins of life. They’d taken gases present on the early Earth like methane, ammonia and hydrogen and applied a spark discharge to them, to mimic lightning. From that, they produced amino acids, the compounds that make up the proteins in all living organisms. It was a stunning discovery. So when I met Stanley, I was hooked. I switched my thesis to work with him. My Ph.D. idea was to move the spark discharge experiment a step forward by studying amino acid stability.
Q. WHY WAS STABILITY IMPORTANT TO UNDERSTAND?
A. Because, in the turbulent environment of the early Earth, if an amino acid had been too unstable it would have decomposed. There would have never been enough of it to make up what we call “the prebiotic soup,” the molecular ingredients of life. So I suggested this one set of reactions that might constrain their decomposition, and then, in the lab, we did an experiment, which worked the first time we tried it. We were able to show some of the conditions that would allow amino acids to exist for longer time periods. And this allowed us to understand what types of amino acids may have been present on the baby Earth.
Q. DO WE KNOW HOW THEY BECAME LIFE?
A. We are closing in on that question. The Earth had to cool down enough for water to appear. Water allows molecules to dissolve and interact, which is why it is essential to life. We do know that we went from simple molecules to more complex molecules and eventually to RNA, which evolved into DNA. This took about a billion years.
The missing piece of the puzzle is that intermediate phase between the amino acids and the RNA phase. We know that RNA is too complex to have arisen out of the simple molecules of the primordial soup. We can surmise that this intermediate form was able to make copies of itself to pass onto the next generation. Over time, mutations occurred and those mutants with survival advantages thrived and on and on until you eventually got to the complex RNA world.
Q. IS THIS SAME PROCESS AT WORK SOMEWHERE IN THE UNIVERSE NOW?
A. The chemistry we see in the lab is universal chemistry. It takes place anywhere you have the ingredients and conditions. We know that it happened, partially, on some of the meteorites that have come to earth. Amino acids have been found inside some of them.
Q. SO WE’RE PROBABLY NOT ALONE?
A. I don’t want to say “we” because people immediately think of something like a human being. But life as we know it — a self-replicating system — is probably not unique to the Earth. Under the right conditions, with the right chemistry, it can happen. There may be simple chemistry happening on Titan, a moon of Saturn. Some people think it could be happening on the satellites of Jupiter. There are compelling reasons to think that Mars was wet when it was young and that the raw materials for life could have been there. We don’t know how far it progressed.
Q. DID YOU SUPPORT FORMER PRESIDENT GEORGE W. BUSH’S PLAN FOR MANNED TRAVEL TO MARS?
A. I’m glad the Obama administration appears to be canceling it. Until we know whether or not life existed on Mars, putting humans there is premature. We’d contaminate the planet. All we’d see is human microbes and refuse.
Q. ON THE OTHER HAND, STEPHEN HAWKING THINKS EXPOSURE TO EXTRATERRESTRIAL LIFE COULD PROVE HAZARDOUS TO EARTHLINGS. “IF ALIENS VISIT US, THE OUTCOME WOULD BE MUCH AS WHEN COLUMBUS LANDED IN AMERICA, WHICH DIDN’T TURN OUT WELL FOR THE NATIVE AMERICANS,” DR. HAWKING RECENTLY WARNED. IS HE ONTO SOMETHING?
A. Dr. Hawking suggests an intriguing possibility, although there are alternative scenarios. Aliens might bring some exotic organisms with them to Earth, but would they survive in our oxygen-rich environment long enough to do any damage to humans or the ecosystem? Meteorites from Mars have landed on Earth throughout history, and if any Martian organisms were hitchhiking on these, they have not killed us yet.
Q. THREE YEARS AGO, YOU REDID THE SPARK DISCHARGE EXPERIMENT. WHY?
A. Actually, we didn’t redo it — we reanalyzed it. When, in 1999, Stanley had a stroke, he donated everything in his office to my laboratory. Eight years later, I was giving a talk in Texas and someone there told me that he’d once seen the extracts of the 1953 experiment in a cardboard box in Stanley’s laboratory. Stanley had kept them for all those decades! Though we were very close, he’d never told me about it. I suppose it was just old business to him. But when I got back to San Diego, I asked my staff, “When we moved everything from Stanley’s office, did we get a little cardboard box?” And someone said, “Yeah, its right over there.”
And there it was! Inside, were all these tiny glass vials carefully labeled, with page numbers referring Stanley’s laboratory notes. I was dumbstruck. We were looking at history. It immediately hit me that when Stanley had first done the experiment, analytic tools were still very, very primitive. We have instruments today that are a billion times better. So we then reanalyzed the original materials with the modern tools. And lo and behold, we found that the spark discharge experiment had actually made about 30 compounds. Stanley had shown they’d made only five!
Q. DID YOUR ANALYSIS CONFIRM THE VALIDITY OF THE EXPERIMENT? THERE ARE SOME PEOPLE WHO STILL DOUBT IT.
A. Oh, yes. And more! It showed how easy it was to make a huge number of compounds. What was really interesting was the Murchison meteorite [ http://www.scientificamerican.com/article.cfm?id=murchison-meteorite ], which fell to earth in 1969, was originally thought to contain maybe 75 amino acids. Three years ago, when we compared the distribution of amino acids in the discharge experiment, they were uncannily similar to those in the meteorite.
I would have loved to have told Stanley about this. But sadly, he was completely incapacitated by the time we found the samples. He died shortly thereafter. I would have loved to have said to him, “Look Stanley, your experiment duplicated what happened on Murchison and you didn’t know it.”
Q. DO YOU THINK IT WOULD BE VALUABLE FOR OTHER SCIENTISTS TO REVISIT OLDER EXPERIMENTS?
A. Listen, I was very fortunate to have had a mentor who had the foresight to preserve his experimental materials. Nonetheless, he couldn’t anticipate all the changes that came with modern analytical chemistry. Today, we are seeing an explosion of knowledge due to new techniques. We ought to use them.
There was just an article in the journal PNAS [ http://www.pnas.org/content/107/7/2763.abstract ] about how new methods further characterized the compounds in Murchison. Up to now, we knew of hundreds in it. Yet, because of new techniques, they’ve found tens of thousands. And that made me think that yes, we ought to redo the spark discharge experiment. I suspect we’d also find tens of thousands of molecules that were in the prebiotic soup. That, hopefully, is the next project we’ll be pursuing.
© 2011 The New York Times Company (emphasis in original)
http://www.nytimes.com/2010/05/18/science/18conv.html
===
The Pre-History of Life: elegantly simple organizing principles seen in ribosomes
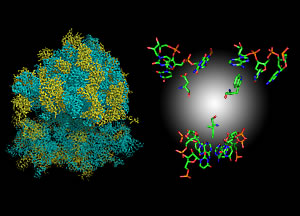
Analysis of ribosome structures (shown on the left) from four different species revealed a non-random affinity between anticodon-containing RNA triplets and their respective amino acids (shown on the right).
Courtesy of David Johnson, Salk Institute for Biological Studies.
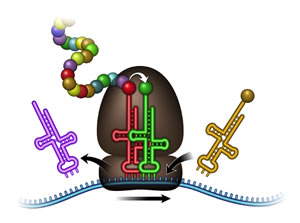
Ribosomes bring together all the mechanical machinery necessary for translation. They couple tRNAs to their proper codons on the mRNA and facilitate the formation of peptide bonds between amino acids.
Image: Courtesy of Jamie Simon, Salk Institute for Biological Studies
April 12, 2010
LA JOLLA, CA—With few exceptions, all known forms of life on our planet rely on the same genetic code to specify the amino acid composition of proteins. Although different hypotheses abound, just how individual amino acids were assigned to specific three-letter combinations or codons during the evolution of the genetic code is still subject to speculation.
Taking their hints from relics of this evolution left behind in modern cells, researchers at the Salk Institute for Biological Studies concluded that after only two waves of "matching" and some last minute fiddling, all 20 commonly used amino acids were firmly linked with their respective codons, setting the stage for the emergence of proteins with unique, defined sequences and properties.
Their findings, which will be published in next week's online edition of the Proceedings of the National Academy of Sciences [actually published the same day, abstract http://www.pnas.org/content/107/18/8298.abstract?sid=06798e39-fe19-48c6-b37c-ea4f230b93ea , full text http://www.pnas.org/content/107/18/8298.full , full text pdf http://www.pnas.org/content/107/18/8298.full.pdf - http://www.pnas.org/content/107/18/8298.full.pdf+html ], provide the first in vivo data shedding light on the origin and evolution of the genetic code.
"Although different algorithms, or codes, were likely tested during a long period of chemical evolution, the modern code proved so robust that, once it was established, it gave birth to the entire tree of life," says the study's lead author Lei Wang [ http://www.salk.edu/faculty/wang.html ], Ph.D., an assistant professor in the Chemical Biology and Proteomics Laboratory.
"But the universality of the code makes it very hard for researchers to study its formation since there are no organisms using a primitive or intermediate genetic code that we could analyze for comparison," he explains.
Cells provide a dazzling variety of functions that cover all of our body's needs, yet they make do with a very limited number of molecular building blocks. With few exceptions, all known forms of life use the same common 20 amino acids—and only those 20—to keep alive organisms as diverse as humans, earthworms, tiny daisies, and giant sequoias.
Each of the 20 amino acids is matched to its own carrier molecule known as transfer RNA (tRNA). During protein synthesis, which is coordinated by so-called ribosomes, amino acids are brought out one by one by their respective tRNAs and inserted in the growing protein chain according to the instructions spelled out in the universal language of life—the genetic code. The code is "read" with the help of anticodons embedded in each tRNA, which pair up with their codon-counterparts.
Several hypotheses have been put forward to explain why codons are selectively assigned to specific amino acids. "One of the theories, the stereochemical hypothesis, gained some traction when researchers could show that short codon- or anticodon-containing polynucleotide molecules like to interact with their respective amino acids," says graduate student and first author David B. F. Johnson.
If chemical or physical interactions between amino acids and nucleotide indeed drove the formation of the genetic code, Johnson reasoned, then he should be able to find relics of this mutual affinity in modern cells. He zoomed in on ribosomes, large complexes consisting of some 50 proteins interacting closely with ribosomal RNAs.
"Also, the ribosome emerged from an early evolutionary stage of life to help with the translation of the genetic code before the last universal common ancestor," explains Wang, "and therefore is more likely to serve as a molecular fossil that preserved biological evidence."
When Wang and Johnson probed bacterial ribosomes for imprints of the genetic code, they found evidence that direct interactions between amino acids and nucleotide triplet anticodons helped establish matching pairs. "We now believe that the genetic code was established in two different stages," says Johnson.
Their data does not shed much light on the early code, consisting of prebiotically available amino acids—the kind generated in Stanley Miller's famous "zap"-experiment. But once some primitive translational mechanism had been established, new amino acids were added to the mix and started infiltrating the genetic code based on specific amino acid/anticodon interactions.
"We found evidence that a few amino acids were reassigned to a different codon but once the code was in place it took over," says Johnson. "It might not have been the best possible solution but the only one that was viable at the time."
The work was supported in part by the Searle Scholar Program, the Beckman Young Investigator Program and the National Institutes of Health Director's New Innovator Award.
*
About the Salk Institute for Biological Studies
The Salk Institute for Biological Studies is one of the world's preeminent basic research institutions, where internationally renowned faculty probe fundamental life science questions in a unique, collaborative, and creative environment. Focused both on discovery and on mentoring future generations of researchers, Salk scientists make groundbreaking contributions to our understanding of cancer, aging, Alzheimer's, diabetes and infectious diseases by studying neuroscience, genetics, cell and plant biology, and related disciplines.
Faculty achievements have been recognized with numerous honors, including Nobel Prizes and memberships in the National Academy of Sciences. Founded in 1960 by polio vaccine pioneer Jonas Salk, M.D., the Institute is an independent nonprofit organization and architectural landmark.
© 2011 Salk Institute for Biological Studies
http://www.salk.edu/news/pressrelease_details.php?press_id=417 [also at e.g. http://esciencenews.com/articles/2010/04/12/the.pre.history.life.elegantly.simple.organizing.principles.seen.ribosomes ]
===
A Simple Solution to Life's Origin

The natural enantiomer of the RNA precursor molecules formed a crystal structure visible to the naked eye.
Credit: UC Merced

Model of an RNA molecule.
Image Credit: Lawrence Berkeley National Laboratory
Summary: A new study shows how a relatively simple combination of naturally occurring sugars and amino acids offers a plausible route to the building blocks of life.
Origin & Evolution of Life
Posted: 08/12/11
Source: UC Merced [ http://www.ucmerced.edu/ ] press release [ http://www.ucmerced.edu/news/study-builds-plausible-scenario-origin-life-earth ]
A relatively simple combination of naturally occurring sugars and amino acids offers a plausible route to the building blocks [ http://astrobiology.nasa.gov/roadmap ] of life, according to a paper published in Nature Chemistry co-authored by a professor at the University of California, Merced.
The study [ http://www.nature.com/nchem/journal/v3/n9/full/nchem.1108.html ], "A Route to Enantiopure RNA Precursors from Nearly Racemic Starting Materials," shows how the precursors to RNA could have formed on Earth before any life existed. It was authored by Jason E. Hein, Eric Tse and Donna G. Blackmond, a team of researchers with the Scripps Research Institute. Hein is now a chemistry professor with UC Merced. The paper was published online Sunday.
Biological molecules, such as RNA and proteins, can exist in either a natural or unnatural form, called enantiomers. By studying the chemical reactions carefully, the research team found that it was possible to generate only the natural form of the necessary RNA precursors by including simple amino acids.
"These amino acids changed how the reactions work and allowed only the naturally occurring RNA precursors to be generated in a stable form," said Hein. "In the end, we showed that an amazingly simple result emerged from some very complex and interconnected chemistry."
The natural enantiomer of the RNA precursor [ http://www.astrobio.net/exclusive/137/unfamiliar-life ] molecules formed a crystal structure visible to the naked eye. The crystals are stable and avoid normal chemical breakdown. They can exist until the conditions are right for them to change into RNA.
The study was led by Blackmond and builds on the work of John D. Sutherland and Matthew W. Powner published in 2009 and covered by outlets such as The New York Times and Wired. Sutherland is a chemist with Cambridge's Medical Research Council Laboratory of Molecular Biology. Powner is a post-doctoral scholar with Harvard University.
Copyright © 2011, Astrobio.net
http://www.astrobio.net/pressrelease/4148/a-simple-solution-to-lifes-origin
===
Hunting The Replicators: How Did Life Begin?

How did this get started?
AJC1 / http://flic.kr/p/XM1U9
by Adam Frank
August 16, 2011 04:25 pm
Amoebas are alive but rocks are not. What is the difference?
For many people, one of the greatest difficulties in understanding the scientific narrative of cosmic evolution is how nature took the path from non-life to life. This question of "abiotic genesis" haunts many a science and religion debate with advocates of scriptural literalism unwilling to grant that natural processes, unmediated by a higher intelligence, could have taken "dead" matter and created living material.
Now the path from "just" molecules to "something more" has gotten a bit more clear as researchers take a crucial step toward building the holy of holies: a self-replicating molecule.
Part of this story, however, is the funny thing that happened to scientists studying the origin of life over the last 80 years. Their perspectives were profoundly rewired.
In the 1950s, chemists Stanley Miller and Harold Urey performed a brilliant and brilliantly illustrative experiment [ http://www.chem.duke.edu/~jds/cruise_chem/Exobiology/miller.html ]. Miller and Urey created a simulated version of the early Earth in a test tube. An "atmosphere" of hydrogen, ammonia and methane was created in one flask. An "ocean" of liquid water was held in a separate flask. The two were connected and a high voltage discharge was set up in the atmospheric flask to act as lightning (and a source of UV light).
After running the experiment for a week, Miller and Urey found a brown goo had formed in their test-tube ocean. When they analyzed the goo they found it formed a rich soup of pre-biotic molecules. Amino acids, sugars and lipids (the basis of fat) had all been generated. The Miller-Urey experiment was hailed as instant classic of biochemistry and proof that, in principle, non-living material along with the natural processes of physics and chemistry could create the molecular basis of life. All that had to be added, scientists argued, was time and then life would have its start.
With time, however, scientists realized that there had to be more to the story of abiotic genesis. While the Miller-Urey experiment was revolutionary, as researchers came to understand the links between the molecular engines of life —DNA, RNA and proteins — it was clear their perspective had to shift. As the astrobiologist [ http://en.wikipedia.org/wiki/Astrobiology ] Chris McKay likes to put it, scientists eventually saw the problem was not just about chemistry but also about information processing as well.
As everyone knows, DNA constitutes the molecular blueprint for life. With the help of the molecule RNA, DNA can be read and transformed into proteins that are the workhorses of life. Proteins can fold up into a remarkable array of shapes giving them the ability to do everything from providing stable cellular structures to catalyzing chemical reactions when, and only when, they are needed.
Obviously, getting life started means getting a self-replicating molecule. That molecule must be capable of storing information about its own structure, operations and, of course, replication. DNA might seem the obvious choice, but it cannot replicate alone. DNA needs RNA and proteins to make copies of itself. That machinery could not have existed on the early Earth. Thus researchers had to find a molecule that could have formed on its own and with its own software.
As far back as 30 years ago RNA, a simpler molecule than DNA, was suggested as a candidate. Once it was recognized that RNA could fold up (like proteins) in ways that led to self-reactions, researchers saw the potential for an "RNA World [ http://www.panspermia.org/rnaworld.htm ]". The idea was that the early Earth must have been awash in RNA molecules building copies of themselves and enhancing their own abilities to store, process and act on information.
A key bottleneck in the process was finding examples of RNA with the "software" to process large sets of instructions and fully replicate themselves. This step is crucial because, for example, if you have a robot that can only build a hundredth of a robot then the dreaded robot apocalypse is probably still a long way away. Likewise, getting that first truly self-replicating molecule is the first true step towards evolution. Scientists have, up till recently, had a hard time finding an example of RNA that had the self-replicating chops.
But, as New Scientist [ http://www.newscientist.com/article/mg21128251.300-first-life-the-search-for-the-first-replicator.html ( http://www.newscientist.com/article/mg21128251.300-first-life-the-search-for-the-first-replicator.html?full=true ) (next below)] reports, researcher Philipp Holliger in Cambridge England has now taken a key step forward. Together with his collaborators, Holliger has created an RNA enzyme called tC19Z that can make copies of molecules almost half its own own length. If this does not sound impressive you need to understand that tC19Z is factor of 5 more capable than previous attempts at self replicating RNA. It's close enough in its ability to make endless copies of itself to make the RNA world seem like nature's best choice for creating life.
While we will never have molecular fossils that can tell us exactly what did happen four billion years ago, our progress in molecular biology is now allowing us to see what likely could have happened. Most importantly these new results are part of the long paradigm shift recognizing that living systems are as much about information processing as they are about moving material around.
Long live the replicators — whatever and wherever they are.
Copyright 2011 NPR (emphasis in original)
http://www.npr.org/blogs/13.7/2011/08/16/139673287/hunting-the-replicators-how-did-life-begin [with comments]
===
First life: The search for the first replicator

Dawn of the living
(Image: Gina and Matt)
Life must have begun with a simple molecule that could reproduce itself – and now we think we know how to make one
by Michael Marshall
15 August 2011
4 BILLION years before present: the surface of a newly formed planet around a medium-sized star is beginning to cool down. It's a violent place, bombarded by meteorites and riven by volcanic eruptions, with an atmosphere full of toxic gases. But almost as soon as water begins to form pools and oceans on its surface, something extraordinary happens. A molecule, or perhaps a set of molecules, capable of replicating itself arises.
This was the dawn of evolution. Once the first self-replicating entities appeared, natural selection kicked in, favouring any offspring with variations that made them better at replicating themselves. Soon the first simple cells appeared. The rest is prehistory.
Billions of years later, some of the descendants of those first cells evolved into organisms intelligent enough to wonder what their very earliest ancestor was like. What molecule started it all?
As far back as the 1960s, a few of those intelligent organisms began to suspect that the first self-replicating molecules were made of RNA, a close cousin of DNA. This idea has always had a huge problem, though - there was no known way by which RNA molecules could have formed on the primordial Earth. And if RNA molecules couldn't form spontaneously, how could self-replicating RNA molecules arise? Did some other replicator come first? If so, what was it? The answer is finally beginning to emerge.
When biologists first started to ponder how life arose, the question seemed baffling. In all organisms alive today, the hard work is done by proteins. Proteins can twist and fold into a wild diversity of shapes, so they can do just about anything, including acting as enzymes, substances that catalyse a huge range of chemical reactions. However, the information needed to make proteins is stored in DNA molecules. You can't make new proteins [ http://www.newscientist.com/article/mg15220578.100-cellular-factories.html ] without DNA, and you can't make new DNA without proteins. So which came first, proteins or DNA?
The discovery in the 1960s that RNA could fold like a protein, albeit not into such complex structures, suggested an answer. If RNA could catalyse reactions as well as storing information, some RNA molecules might be capable of making more RNA molecules. And if that was the case, RNA replicators would have had no need for proteins. They could do everything themselves.
It was an appealing idea, but at the time it was complete speculation. No one had shown that RNA could catalyse reactions like protein enzymes. It was not until 1982, after decades of searching, that an RNA enzyme was finally discovered. Thomas Cech of the University of Colorado in Boulder found [ http://www.newscientist.com/article/mg12416871.900-prizewinners-came-by-separate-paths-to-understand-rna.html ] it in Tetrahymena thermophila [ http://www.newscientist.com/article/dn20191-zoologger-the-hairy-beast-with-seven-fuzzy-sexes.html ], a bizarre single-celled animal with seven sexes (Science, vol 231, p 4737 [ http://www.sciencemag.org/content/231/4737/470 ]).
After that the floodgates opened. People discovered ever more RNA enzymes in living organisms and created new ones in their labs. RNA might be not be as good for storing information as DNA, being less stable, nor as versatile as proteins, but it was turning out to be a molecular jack of all trades. This was a huge boost to the idea that the first life consisted of RNA molecules that catalysed the production of more RNA molecules - "the RNA world", as Harvard chemist Walter Gilbert dubbed it 25 years ago (Nature, vol 319, p 618 [ http://www.nature.com/nature/journal/v319/n6055/abs/319618a0.html ]).
These RNA replicators may even have had sex. The RNA enzyme Cech discovered did not just catalyse any old reaction. It was a short section of RNA that could cut itself out of a longer chain [ http://www.newscientist.com/article/mg13217944.500-the-first-gene-on-earth.html ]. Reversing the reaction would add RNA to chains, meaning RNA replicators might have been able to swap bits with other RNA molecules. This ability would greatly accelerate evolution, because innovations made by separate lineages of replicators could be brought together in one lineage.
Evolving replicators
For many biologists the clincher came in 2000, when the structure of the protein-making factories [ http://www.newscientist.com/article/mg16722521.800-factory-of-life-gives-up-its-secrets.html ] in cells was worked out. This work confirmed that nestling at the heart of these factories is an RNA enzyme [ http://www.newscientist.com/article/dn17933-nobel-for-explaining-natures-protein-factories.html ] - and if proteins are made by RNA, surely RNA must have come first.
Still, some issues remained. For one thing, it remained unclear whether RNA really was capable of replicating itself. Nowadays, DNA and RNA need the help of many proteins to copy themselves. If there ever was a self-replicator, it has long since disappeared. So biochemists set out to make one, taking random RNAs and evolving them for many generations to see what they came up with.
By 2001, this process had yielded an RNA enzyme called R18 that could stick 14 nucleotides - the building blocks of RNA and DNA - onto an existing RNA, using another RNA as a template (Science, vol 292, p 1319 [ http://www.sciencemag.org/content/292/5520/1319 ]). Any self-replicating RNA, however, needs to build RNAs that are at least as long as itself - and R18 doesn't come close. It is 189 nucleotides long, but the longest RNA it can make contains just 20.
A big advance came earlier this year, when Philipp Holliger of the MRC Laboratory of Molecular Biology in Cambridge, UK, and colleagues unveiled an RNA enzyme called tC19Z [ http://www.newscientist.com/blogs/shortsharpscience/2011/04/rna-enzyme-makes-another-rna-e.html ]. It reliably copies RNA sequences up to 95 letters long, almost half as long as itself (Science, vol 332, p 209 [ http://www.sciencemag.org/content/332/6026/209 ]). To do this, tC19Z clamps onto the end of an RNA, attaches the correct nucleotide, then moves forward a step and adds another. "It still blows my mind that you can do something so complex with such a simple molecule," Holliger says.
So biologists are getting tantalisingly close to creating an RNA molecule, or perhaps a set of molecules, capable of replicating itself. That leaves another sticking point: where did the energy to drive this activity come from? There must have been some kind of metabolic process going on - but RNA does not look up to the job of running a full-blown metabolism.
"There's been a nagging issue of whether RNA can do all the chemistry," says Adrian Ferré-D'Amaré of the National Heart, Lung and Blood Institute in Bethesda, Maryland. RNA has only a few chemically active "functional groups", which limit it to catalysing just a few types of chemical reaction.
Functional groups are like tools - the more kinds you have, the more things you can do. Proteins have many more functional groups than RNAs. However, there is a way to make a single tool much more versatile: attach different bits to it, like those screwdrivers that come with interchangeable heads. The chemical equivalents are small helper molecules known as cofactors.
Proteins use cofactors to extend even further the range of reactions they can control. Without cofactors, life as we know it couldn't exist, Ferré-D'Amaré says. And it turns out that RNA enzymes can use cofactors too.
In 2003, Hiroaki Suga, now at the University of Tokyo, Japan, created an RNA enzyme that could oxidise alcohol, with help from a cofactor called NAD+ which is used by many protein enzymes (Nature Structural Biology, vol 10, p 713 [ http://www.nature.com/nsmb/journal/v10/n9/full/nsb964.html ]). Months later, Ronald Breaker of Yale University found that a natural RNA enzyme, called glmS, also uses a cofactor [ http://www.pnas.org/content/101/17/6421 ].
Many bacteria use glmS, says Ferré-D'Amaré, so either it is ancient or RNA enzymes that use cofactors evolve easily. Either way, it looks as if RNA molecules would have been capable of carrying out the range of the reactions needed to produce energy.
So the evidence that there was once an RNA world is growing ever more convincing. Only a few dissenters remain [ http://www.newscientist.com/article/mg15120373.900-let-there-be-life.html ]. "The naysayers about the RNA world have lost a lot of ground," says Donna Blackmond of the Scripps Research Institute in La Jolla, California. But there is still one huge and obvious problem: where did the RNA come from in the first place?
RNA molecules are strings of nucleotides, which in turn are made of a sugar with a base and a phosphate attached. In living cells, numerous enzymes are involved in producing nucleotides and joining them together, but of course the primordial planet had no such enzymes. There was clay, though. In 1996, biochemist Leslie Orgel showed that when "activated" nucleotides - those with an extra bit tacked on to the phosphate - were added to a kind of volcanic clay, RNA molecules up to 55 nucleotides long formed (Nature, vol 381, p 59 [ http://www.nature.com/nature/journal/v381/n6577/abs/381059a0.html ]). With ordinary nucleotides the formation of large RNA molecules would be energetically unfavourable, but the activated ones provide the energy needed to drive the reaction.
This suggests that if there were plenty of activated nucleotides on the early Earth, large RNA molecules would form spontaneously. What's more, experiments simulating conditions on the early Earth and on asteroids show that sugars, bases and phosphates [ http://www.newscientist.com/article/dn14966-volcanic-lightning-may-have-sparked-life-on-earth.html ] would arise naturally too. It's putting the nucleotides together that is the hard bit; there does not seem to be any way to join the components without specialised enzymes. Because of the shapes of the molecules, it is almost impossible for the sugar to join to a base, and even when it does happen, the combined molecule quickly breaks apart.
This apparently insurmountable difficulty led many biologists to suspect to RNA was not the first replicator after all. Many began exploring the possibility that the RNA world was preceded by a TNA world, or a PNA world, or perhaps an ANA world. These are all molecules similar to RNA but whose basic units are thought to have been much more likely to form spontaneously. The big problem with this idea is that if life did begin this way, no evidence of it remains. "You don't see a smoking gun," says Gerald Joyce, also of the Scripps Research Institute.
In the meantime John Sutherland, at the MRC Laboratory of Molecular Biology, has been doggedly trying to solve the nucleotide problem. He realised that researchers might have been going about it the wrong way. "In each nucleotide, you see a sugar, a base and a phosphate group," he says. "So you assume you need to make those building blocks first and then stick them together... and it doesn't work."
Instead he wondered whether simpler molecules might assemble into a nucleotide without ever becoming sugars or bases. In 2009, he proved it was possible. He took half a sugar and half a base, and stuck them together - forming the crucial sugar-base link that everyone had struggled with. Then he bolted on the rest of the sugar and base. Sutherland stuck on the phosphate last, though he found that it needed to be present in the mixture for the earlier reactions to work (Nature, vol 459, p 239 [ http://www.nature.com/nature/journal/v459/n7244/full/nature08013.html ]).
Goldilocks chemistry
Sutherland was being deliberately messy by including the phosphate from the start, but it gave the best results. That's encouraging: the primordial Earth was a messy place and it may have been ideal for making nucleotides. Sutherland now suspects there is a "Goldilocks chemistry" [ http://www.newscientist.com/article/mg20928023.900-primordial-pacman-oil-droplet-hints-at-lifes-origin.html ] - not too simple, not too complex - that would produce many key compounds from the same melting pot.
"Sutherland had a real breakthrough," Holliger says. "Everyone else was barking up the wrong tree."
The issue isn't entirely solved yet. RNA has four different nucleotides, and so far Sutherland has only produced two of them. However, he says he is "closing in" on the other two. If he succeeds, it will show that the spontaneous formation of an RNA replicator is not so improbable after all, and that the first replicator was most likely made of RNA.
Many questions remain, of course. Where did the first replicators arise? What was the first life like? How did the transition to DNA and proteins, and the development of the genetic code, occur? We may never know for sure but many promising avenues are being explored. Most biologists think there must have been something like a cell right from the start, to contain the replicator and keep its component parts together. That way, individuals could compete for resources and evolve in different ways.
Jack Szostak of Harvard University has shown that the same clay that produces RNA chains also encourages the formation of membrane-bound sacs [ http://www.newscientist.com/article/dn4307-clays-matchmaking-could-have-sparked-life.html ] rather like cells that enclose cells. He has grown "proto-cells" that can carry RNA and even divide [id.] without modern cellular machinery.
Another idea is that life began in alkaline hydrothermal vents on the sea floor [ http://www.newscientist.com/article/mg20427306.200-was-our-oldest-ancestor-a-protonpowered-rock.html ]. Not only are these vents laced with pores and bubbles, but they also provide the same kind of electrochemical gradient that drives energy production in cells to this day. Conditions may have been ideal for producing long RNA chains.
Holliger has a rather surprising idea: maybe it all happened in ice. At the time life began, the sun was 30 per cent dimmer than today. The planet would have frozen over if the atmosphere hadn't been full of greenhouse gases, and there may well have been ice towards the poles.
Cold RNA lasts longer, and ice has many other benefits. When water laced with RNA and other chemicals is cooled, some of it freezes while the rest becomes a concentrated brine running around the ice crystals. "You get little pockets within the ice," Holliger says. Interestingly, the R18 RNA enzyme works better in ice than at room temperature (Nature Communications, DOI: 10.1038/ncomms1076 [ http://www.nature.com/ncomms/journal/v1/n6/full/ncomms1076.html ]).
Right now, there's no way to choose between these options. No fossilised vestiges remain of the first replicators as far as we know. But we can try recreating the RNA world to demonstrate how it might have arisen. One day soon, Sutherland says, someone will fill a container with a mix of primordial chemicals, keep it under the right conditions, and watch life emerge. "That experiment will be done."
Michael Marshall is a reporter for New Scientist
© Copyright Reed Business Information Ltd.
http://www.newscientist.com/article/mg21128251.300-first-life-the-search-for-the-first-replicator.html [ http://www.newscientist.com/article/mg21128251.300-first-life-the-search-for-the-first-replicator.html?full=true ] [with comments]
===
‘It’s Alive! It’s Alive!’ Maybe Right Here on Earth
Video [embedded]
Life Out There: Eden in a Test Tube

To better recognize extraterrestrial life should they come upon it, scientists are working to create simple life forms in a lab. But, as Dennis Overbye reports, they first have to agree what life is.
Jim Wilson/The New York Times


Dr. Joyce in his lab at the Scripps Research Institute in San Diego.
Jim Wilson/The New York Times
By DENNIS OVERBYE
Published: July 27, 2011
SAN DIEGO — Here in a laboratory perched on the edge of the continent, researchers are trying to construct Life As We Don’t Know It in a thimbleful of liquid.
Generations of scientists, children and science fiction fans have grown up presuming that humanity’s first encounter with alien life will happen in a red sand dune on Mars, or in an enigmatic radio signal from some obscure star.
But it could soon happen right here on Earth, according to a handful of chemists and biologists who are using the tools of modern genetics to try to generate the Frankensteinian spark that will jump the gap separating the inanimate and the animate. The day is coming, they say, when chemicals in a test tube will come to life.
By some measures, Gerald F. Joyce, a professor at the Scripps Research Institute here, has already crossed that line, although he would be the first to say he has not — yet.
Biologists do not agree on what the definition of life should be or whether it is even useful to have one. But most do agree that the ability to evolve and adapt is fundamental to life. And they also agree that having a second example of life could provide insight to how it began and how special life is or is not in the universe, as well as a clue for how to recognize life if and when we do stumble upon it out there among the stars.
“Everything we know about life is based on studies of life on Earth,” said Chris McKay, a researcher at NASA’s Ames Research Laboratory in Mountain View, Calif.
Dr. Joyce said recently: “It drives me crazy when astronomers say, ‘Surely the universe is pregnant with life.’ If we have an Earthlike planet, what are the chances of life arising? Is it one in a million? Is it one in two? I don’t see how you can say.”
He continued, “If you had a second example of life, even if it were synthetic, you might know better. I’m betting we’re just going to make it.”
Four years ago Dr. Joyce and a graduate student, Tracey A. Lincoln, now a researcher at the University of Massachusetts Medical School, evolved a molecule in a test tube that could replicate and evolve all by itself, swapping little jerry-built genes in a test tube forever, as long as it was supplied with the right carefully engineered ingredients.
An article in the Joyce Laboratory newsletter called it “The Immortal Molecule.” Dr. Joyce’s molecule is a form of RNA, or ribonucleic acid, which plays Robin to DNA’s Batman in Life As We Do Know It, assembling proteins in accordance with the blueprint encoded in DNA. Neither RNA nor DNA is alive by itself, any more than any other chemical, like bleach, or a protein. But in Dr. Joyce’s test tube, his specially engineered RNA molecule comes close, copying itself over and over, and evolving.
But, Dr. Joyce says, “We really would hope for more from our molecules than just replicating.”
Reproduction is the job of any life, he explained, but Earthly organisms have evolved a spectacular set of tricks to improve the odds of success — everything from peacock feathers to whale songs. Dr. Joyce’s molecules have not yet surprised him by striking out on their own to invent the molecular equivalent of writing a hit pop song.
It is only a matter of time, he said, before they do.
“Our job is to give them the running room to do that,” Dr. Joyce said.
The deeper philosophical and intellectual ramifications of test tube life are as enormous as they are unknown. The achievement would probably not come with sci-fi drama, say scientists who are squeamish about such matters anyway, saying such speculation is beyond their pay grade. No microbe is going to leap out of the Petri dish and call home, or turn the graduate students into zombies. Indeed, given the human penchant for argument and scientists’ habit of understatement, it could be years before everybody agrees it has been done.
“The ability to synthesize life will be an event of profound importance, like the invention of agriculture or the invention of metallurgy,” Freeman Dyson, a mathematician and physicist at the Institute for Advanced Study in Princeton, wrote in an e-mail. “Nobody can tell in advance what will come of it.”
On Earth, all life as we know it is based on DNA, the carbon-based molecule that contains the instructions for making and operating living cells in a four-letter alphabet along its double-helix spine.
The possibilities of a second example of life are as deep as the imagination. It could be based on DNA that uses a different genetic code, with perhaps more or fewer than four letters; it could be based on some complex molecule other than DNA, or more than the 20 amino acids from which our own proteins are made, or even some kind of chemistry based on something other than carbon and the other elements that we take for granted, like phosphorous or iron. Others wonder whether chemistry is necessary at all. Could life manifest itself, for example, in the pattern of electrically charged dust grains in a giant interstellar cloud, as the British astronomer and author Fred Hoyle imagined in his novel “The Black Cloud”?
Dr. Joyce said that his RNA replicators would count as such a “second example, albeit one constructed as a homage to our ancient ancestors.”
So far, he said, his work with Dr. Lincoln has shown that manmade molecules can evolve over successive generations. “They can pass information from parent to progeny, they can mutate,” Dr. Joyce said. “They can win or die. The molecules are doing it all. We’re just keeping the lights on.”
Dr. Joyce’s molecules may not be clever enough yet to qualify as life in his view, but all sorts of alternatives are being explored in other labs.
Some researchers, like Steven Benner of the Foundation for Applied Molecular Evolution in Florida, are constructing and experimenting with forms of DNA that use coding alphabets of more than four letters. J. Craig Venter, who helped spearhead the decoding of the human genome and now works as president of the J. Craig Venter Institute, recently used store-bought chemicals to reconstruct the genome of a bacterial goat parasite and put it in another bacterium, where it took over, churning out copies of itself with Dr. Venter’s watermark inscribed in its gene code.
In a related vein, George Church and Farren Isaacs of the Harvard Medical School recently reported that they had reprogrammed the genome of an E. Coli bacterium [ http://www.nytimes.com/2011/07/15/health/15genome.htm ], opening up the possibility of incorporating new features into the ubiquitous little bug. Dr. Joyce called the work “really macho molecular biotechnology.”
Jack Szostak of Harvard Medical School and his collaborators have embarked on an ambitious project to build an artificial cell that can replicate and presumably evolve. Dr. Benner wrote in an e-mail, “In my view, a terran laboratory will make synthetic life before NASA or the E.S.A. finds it elsewhere,” referring to the European Space Agency. He added, “And a lot before, given the disassembling of NASA by the current administration.”
According to modern science, life on Earth originated about 3.8 billion years ago, perhaps in a warm pond, as Darwin speculated, or perhaps in a boiling, bubbling mud bath or a scorching volcanic vent way under the sea. The first inhabitant of this Eden, chemists suspect, was RNA.
In today’s world RNA runs errands for DNA. Like DNA, RNA encodes genetic information. Unlike DNA, however, RNA can also catalyze chemical reactions between other molecules, chopping them up or binding them together, a task mostly performed by proteins in modern organisms.
In 1962, the M.I.T. biologist Alexander Rich suggested that RNA could have played both roles — blueprint and machinery — at the beginning. Scientists cannot prove that this is how life arose on Earth, but they can do the next best thing. They can make their own RNA and see if they can then breathe life into it.
Enter Dr. Joyce, who says he came to his vocation by reading “Gravity’s Rainbow,” Thomas Pynchon’s 1973 novel about rockets and death in World War II, while he was a student at the University of Chicago. The last section of that book, he pointed out, is called “The Counterforce,” about pockets of life and love carving order out of the rubble of wartime Europe. For biologists the counterforce creating order and life out of chaos is simply Darwinian evolution, Dr. Joyce explained. “I wanted to be a member of the counterforce.”
At the center of the Joyce lab experiments is a T-shaped piece of RNA that has the ability to glue together other molecules of RNA. In 2002, Dr. Joyce and a postdoctoral fellow, Natasha Paul, configured it to recognize and glue together a pair of smaller molecules, essentially an L and a straight piece. When joined, those molecules would form a new copy of the original T-shaped molecule. It worked; the RNA was able to manufacture new versions of itself, but not fast enough to keep up with the original RNA’s natural tendency to fall apart. Essentially it was dying faster than it was reproducing. Dr. Joyce and Dr. Lincoln found a way to speed the process up, by having two complementary versions of the RNA manufacture each other.
“There was a day that it all happened,” said Dr. Joyce, namely Oct. 1, 2007, when as he puts it, the replicators “went critical,” and their population began growing exponentially.
The game, as he likes to say, was on. And it has never stopped. Dr. Joyce and his colleagues next proceeded to engineer a sort of March Madness for molecules. They synthesized 12 versions of the replicators, which could mutate and evolve to improve their ability to reproduce. The experimenters threw these into the pot, along with the appropriate “food” segments, to compete. “They just go at it,” Dr. Joyce explained.
By the end, the winning molecules were doubling their numbers every 15 minutes. Mistaken swaps had produced combinations, mutations, that had not been in the mix at the start. Most of the original versions almost completely disappeared. In short, the molecule evolved.
“Evolution is not a theory for us chemists,” Dr. Joyce said. “It’s what molecules do when they have the property to replicate and transmit information from parents to progeny.”
In a separate experiment the molecules were redesigned so that they would replicate only when another chemical was present. “That’s the app that’s going to pay for this,” said Dr. Joyce, explaining that the replicating molecules could be fashioned into sensors to detect pollutants or dangerous toxins in the environment. Dr. Joyce and his collaborators are now starting to run the same tournament with 256 versions of the replication enzyme. “We are pipetting madly,” he reported recently.
That means that there will be about 65,000 possible gene combinations that can emerge and try out their wings, which means things are getting interesting. As Dr. Lincoln said, “We’re knocking on the door, but we’re not quite there yet.” Sidney Altman [ http://www.yale.edu/mcdb/facultystaff/altman.html ], a Yale professor who shared a Nobel prize for discovering some of the talents of RNA, said that true test tube life could still be years away. “Gerry Joyce’s replicators are very clever molecules,” he said, but added that they were not self-sufficient enough to be alive.
Dr. Joyce said his team was working on having the replicating molecule invent a new ability, but he would not say what it was. Asked for an example of the kind of things he could teach his RNA to do, Dr. Joyce suggested it could take part in creating one of the ingredients for its own replication by adding together a pair of smaller molecules. “What would be cool,” he said, “would be if they could make their own food.” The key to more ability, he said, is complexity. His molecule has only two genes, compared with 25,000 in human beings, and experiments involved fiddling with four letters of these genes. The human genome has three billion letters.
“We have a little toy genome where we can have the complete book of life,” he said, “but the sentences only have two words.”
Dr. Joyce’s molecules will never catch up to the biosphere. But someday their genome may surprise their creator with a word — a trick or a new move in the game of almost life — that he has not anticipated. “If it would happen, it would do it for me, I would be happy,” Dr. Joyce said, adding, “I won’t say it out loud, but it’s alive.”
*
Life Out There
From Inanimate to Animate
Articles in this series examine the search for new forms of life in the universe.
http://topics.nytimes.com/top/news/science/series/life_out_there/index.html
*
© 2011 The New York Times Company
http://www.nytimes.com/2011/07/28/science/28life.html [ http://www.nytimes.com/2011/07/28/science/28life.html?pagewanted=all ] [comments at http://community.nytimes.com/comments/www.nytimes.com/2011/07/28/science/28life.html ]
===
Amino Acid Alphabet Soup

The amino acid sequence of a protein determines the higher levels of structure of the molecule. A single change in the amino acid sequence can have profound biological effects on the overall structure and function of the protein.
Image Credit: IACR

Amino acids have been found in meteorites, such as the Murchison meteorite.
Image Credit: NASA

How did life end up selecting which amino acids in the primordial soup worked best?
Image Credit: Peter Sawyer / Smithsonian Institution
[image from http://www.space.com/12665-alien-life-chemical-code-amino-acids.html ]

Comet Wild 2 imaged just after flyby. Samples of the gas and dust coming off of the comet collected by the Stardust spacecraft contained the amino acid glycine.
Credit: NASA/JPL

Astronomers have detected hydrogen cyanide and methanimine, two carbon compounds that can combine with water to make the amino acid glycine, in the Arp 220 galaxy.
Image Credit: NASA, ESA, and C. Wilson (McMaster University, Hamilton, Ontario, Canada)
[image from http://www.space.com/12665-alien-life-chemical-code-amino-acids.html ]
Summary: All life on Earth relies on a standard set of 20 amino acids to build the proteins that carry out life's essential actions. But did it have to be this way?
Origin & Evolution of Life
Posted: 08/18/11
Author: Clara Moskowitz
All life on Earth relies on a standard set of 20 molecules called amino acids to build the proteins that carry out life's essential actions. But did it have to be this way?
All living creatures on this planet use the same 20 amino acids, even though there are hundreds available in nature. Scientists therefore have wondered if life could have arisen based on a different set of amino acids. And what's more, could life [ http://astrobiology.nasa.gov/roadmap ] exist elsewhere that utilizes an alternate collection of building blocks?
"Life has been using a standard set of 20 amino acids to build proteins for more than 3 billion years," said Stephen J. Freeland of the NASA Astrobiology Institute at the University of Hawaii. "It's becoming increasingly clear that many other amino acids were plausible candidates, and although there's been speculation and even assumptions about what life was doing, there's been very little in the way of testable hypotheses."
So Freeland and his University of Hawaii colleague Gayle K. Philip devised a test to try to learn if the 20 amino acids Earth's life uses were randomly chosen, or if they were the only possible ones that could have done the job. Amino acids are molecules built primarily from carbon, hydrogen, oxygen, and nitrogen. They assemble in particular shapes and patterns to form larger molecules called proteins that carry out biological functions.
"Technically there is an infinite variety of amino acids," Freeland told Astrobiology Magazine. "Within that infinity there are lots more than the 20 that were available [when life originated on Earth] as far as we can tell."
Testing the possibilities
The researchers defined a likely pool of candidate amino acids from which life drew its 20. They started with the amino acids that have been discovered within the so-called Murchison meteorite, a space rock that fell in Murchison, Victoria in Australia in September 1969. The rock is thought to date from the early solar system, and to represent a sample of which compounds existed in the solar system and on Earth before life began.
The scientists then used computers to estimate the fundamental properties of the 20 amino acids life uses, such as size, charge and hydrophilicity, or the extent to which the molecules are attracted to water.
"We know that these three are important to the ways they build proteins," Freeland said.
Freeland and Philip analyzed whether these properties could have been achieved with as much coverage and efficiency with other combinations of 20 amino acids.
The researchers discovered that life seemingly did not choose its 20 building blocks randomly. "We found that chance alone would be extremely unlikely to pick a set of amino acids that outperforms life's choice," Freeland said.
Natural selection
In fact, the researchers think early life on Earth probably used a version of natural selection to choose these amino acids. Some combinations of other amino acids were likely tried, but none proved quite as fit, so no other combinations ended up producing the numbers of successful offspring that the existing set achieved.
"Here we found a very simple test that begins to show us that life knew exactly what it was doing," Freeland said. "This is consistent with the idea that there was natural selection going on."
Getting at the question of why nature chose the 20 amino acids it did is experimentally difficult, said Aaron Burton, a NASA Postdoctoral Program Fellow who works as an astrochemist at NASA's Goddard Space Flight Center in Greenbelt, Md.
"Although a number of experiments [ http://www.astrobio.net/pressrelease/979/expanding-the-genetic-code ] have shown that unnatural amino acids can be incorporated into the genetic alphabet of organisms, it may never be possible to experimentally simulate sufficient evolutionary time periods to truly compare alternate amino acid alphabets," said Burton, who was not involved in the new study. "As a result, studies such as those presented by Philip and Freeland offer interesting insights and provide a framework for formulating hypotheses that can actually be tested in the lab."
Amino acids in meteorites
Right now the race is on to directly find amino acids elsewhere in the solar system. Some hints that they abound have been found on meteorites that have landed on Earth from outer space, as well as from missions such as NASA's Stardust probe, which sampled the coma [ http://www.astrobio.net/pressrelease/3220/glycine-in-the-grid ] of comet Wild 2 in 2004.
"All signs are that amino acids are going to be found throughout the galaxy," Freeland said. "They are apparently obvious building blocks with which to construct life. What we're finding hints at a certain level of predictability in the way things turned out."
The question of life's amino acid toolbox is interesting not just in trying to trace the origin of the life on Earth, but in wondering whether life exists on other planets, and if so, what form it takes. Scientists are particularly curious about how a different set of amino acid building blocks would result in different characteristics in the life it creates.
"That is the biggest question of all," Freeland said. "We're trying to find a way to ask, if you change the set of amino acids with which we're building, what effect does that have on the proteins you can build. The most interesting thing is, nobody knows."
Philip and Freeland reported their findings in a paper published [ http://www.liebertonline.com/doi/abs/10.1089/ast.2010.0567 ] in the April 19 issue of the journal Astrobiology.
Copyright © 2011, Astrobio.net
http://www.astrobio.net/exclusive/4161/amino-acid-alphabet-soup
===
How Simple Life Got Complicated?

Studying yeast cells has provided many scientific advancments in biology over the years. Yeast has even made it into space. Above are scanning electron micrographs of Saccharomyces cerevisiae (yeast) grown in microgravity (A) and gravity (B).
Credit: NASA/JSC

Yeast is most famous for its fermentative abilities, which are essential in the production of wine and many other alcoholic beverages. Now, the microorganism is helping scientists understand the development of multicellular life.
Summary: New research shows how single-celled organisms may have initially banded together into multicellular groups.
Origin & Evolution of Life
Posted: 08/13/11
Source: Public Library of Science press release [ http://www.eurekalert.org/pub_releases/2011-08/plos-ith080311.php ]
A new study has created an analog of what researchers think the first multicellular cooperation might have looked like, showing that yeast [ http://www.astrobio.net/interview/367/hitch-hikers-guide-to-biologys-second-datum ] cells—in an environment that requires them to work for their food—grow and reproduce better in multicellular clumps than singly.
A team of researchers, led by Harvard professor Andrew Murray, found that cells of brewer's yeast [ http://www.astrobio.net/pressrelease/3970/a-prehistoric-microbial-world-in-a-glass-of-wine ] that clumped together were able more effectively to manipulate and absorb sugars in their environment than were similar cells that lived singly. The experiments showed that in environments where the yeast's sugar food source is dilute and the number of cells is small, the ability to clump together allowed cells that otherwise would have remained hungry and static to grow and divide.
The work, published August 9 [ http://www.plosbiology.org/article/info%3Adoi%2F10.1371%2Fjournal.pbio.1001122 ] in the online, open access journal PLoS Biology, used the yeast Saccharomyces cerevisiae [ http://www.astrobio.net/pressrelease/979/expanding-the-genetic-code ], which is commonly used in brewing and bread-making and has long been used by scientists as a model organism for understanding single-celled life. Murray and colleagues devised a series of experiments that presented two problems for the yeast cells to solve if they were to take in enough food to grow and divide: the first was how to change their food from an unusable form to a usable form; the second was how to actually take in this food.
The researchers put the yeast in a solution of sucrose—plain old table sugar—which is composed of two simpler sugars, glucose and fructose. Yeast lives on sugar, but the sucrose can't get through the membrane that surrounds the cell. So the yeast makes an enzyme called invertase to chop the sucrose into glucose and fructose, each of which can enter the cell using gate-keeping molecules, called transporters, that form part of the membrane.
The second problem was how to get the glucose and fructose from the place where they were split apart by invertase to the transporters in the cell membrane. The only way to bridge the gap is through diffusion, an inefficient process. Researchers calculated that once a cell makes invertase and chops the larger sugar down to usable bits, only one sugar molecule in 100 would be captured by the cell that made it.
They also calculated that, working alone, a single yeast cell in a dilute solution of sucrose would never take in enough glucose and fructose to be able to grow and divide. But by cooperating, clumps of yeast in that same solution might have a chance. With several cells in proximity, all releasing invertase to create smaller sugars, these cooperating yeast cells [ http://www.astrobio.net/pressrelease/2598/making-artificial-bacteria ] would increase the density of those sugars near the clump, increasing the chances that each cell could take in enough to grow and divide. Sure enough, when the researchers tested these hypotheses on two strains of yeast, they found that the strain which clumped cells together was growing and dividing, while the yeast cells living alone were not.
Murray said the work offers one explanation as to why single-celled organisms might have initially banded together deep in the history of life [ http://astrobiology.nasa.gov/roadmap ], although it's impossible to prove conclusively that this is what happened. "Because there is an advantage to sticking together under these circumstances, and because we know that lots of single-celled organisms make enzymes to liberate goods from their environment, this may be the evolutionary force that led to multicellularity," Murray said. Although, he continued, "short of inventing time travel and going back several billion years to see if this is how it happened…this is going to remain speculation."
Copyright © 2011, Astrobio.net
http://www.astrobio.net/pressrelease/4150/how-simple-life-got-complicated
===
(linked in):
http://investorshub.advfn.com/boards/read_msg.aspx?message_id=64150018 and preceding and following
from earlier/elsewhere this string, http://investorshub.advfn.com/boards/read_msg.aspx?message_id=66812099 and preceding and following, and http://investorshub.advfn.com/boards/read_msg.aspx?message_id=66795926 and preceding and following
http://investorshub.advfn.com/boards/read_msg.aspx?message_id=44715115 and preceding (and any future following)
http://investorshub.advfn.com/boards/read_msg.aspx?message_id=66175494 (and preceding) and following (in particular http://investorshub.advfn.com/boards/read_msg.aspx?message_id=66343804 )
http://investorshub.advfn.com/boards/read_msg.aspx?message_id=66819316 and preceding (and any future following)
Join the InvestorsHub Community
Register for free to join our community of investors and share your ideas. You will also get access to streaming quotes, interactive charts, trades, portfolio, live options flow and more tools.