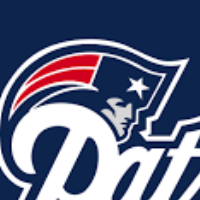
Tuesday, January 29, 2008 12:58:45 PM
I Know it's old info. thought I'd post it anyway.
TRUMEM
http://www.wmsym.org/abstracts/2003/pdfs/258.pdf
APPLICATION OF MEMBRANE SORPTION REACTOR TECHNOLOGY FOR LRW MANAGEMENT
Yuri Glagolenko, Evgeny Dzekun
FSUE "Mayak", Russia
Boris Myasoedov
Institute of Geochemistry and Analytical Chemistry of the Russian Academy of Sciences
Vladimir Gelis, Evgeny Kozlitin and Vitaly Milyutin
Institute of Physical Chemistry of the Russian Academy of Sciences
Lev Trusov
Association "Aspect", Russia
Vadim Tarassov
"Aspect USA", USA
Mike Rengel, Stewart M. Mackay and Michael E. Johnson
CH2M HILL, USA
ABSTRACT
A new membrane-sorption technology has been recently developed and industrially implemented in Russia for the
treatment of the Liquid (Low-Level) Radioactive Waste (LRW).
The first step of the technology is a precipitation of the radionuclides and/or their adsorption
onto sorbents of small particle size. The second step is filtration of the precipitate/sorbent
through the metal-ceramic membrane, Trumem. The unique feature of the technology is a
Membrane-Sorption Reactor (MSR), in which the precipitation / sorption and the filtration of the
radionuclides occur simultaneously, in one stage. This results in high efficiency, high
productivity and compactness of the equipment, which are the obvious advantages of the
developed technology. Two types of MSR based on Flat Membranes device and Centrifugal
Membrane device were developed. The advantages and disadvantages of application of each type
of the reactors are discussed.
The MSR technology has been extensively tested and efficiently implemented at "Mayak " nuclear facility near
Chelyabinsk, Russia as well as at other Russian sites. The results of this and other applications of the MSR
technology at the different Russian nuclear facilities are discussed. The results of the first industrial applications of
the MSR technology for radioactive waste treatment in Russia and analysis of the available information about LRW
accumulated in other countries imply that this technology can be successfully used for the Low Level Radioactive
Waste treatment in the USA and in other nuclear countries.
INTRODUCTION
Modern technologies for the reprocessing of spent nuclear fuel from power nuclear reactors generate substantial
quantities of the liquid radioactive waste (LRW) of different radioactivity level and complex chemical composition.
Taking into account the LRW accumulated up to date as a result of defense programs, the gross radionuclide
WM’03 Conference, February 23-27, 2003, Tucson, AZ
Page - 2 -
quantity currently in storage at Russian nuclear complexes and abroad is about 109 Curies. The most toxic
radionuclides contained in the waste are the long-lived 137Cs, 90Sr, 235U, 239Pu, and 241,243Am, with up to 80% of the
radioactivity being accounted for by the 137Cs radionuclide.
Several approaches used for the LRW treatment and conditioning are as follows:
• tank storage;
• deep injection;
• LRW treatment aimed at the separation of the long-lived radionuclides followed by conversion of the
separated fractions into low soluble compounds.
Tank storage is the simplest way, however, is has the significant drawbacks associated with deterioration of the tank
over time and possible release of radionuclides into the environment. In the case of deep injection of radioactive
waste into the geological collector layers, the monitoring of the injected waste behavior is very complicated.
Long-lived radionuclide separation technology currently employs the following techniques and combinations
thereof:
• extraction processes;
• sorption processes;
• precipitation processes.
An extraction technique using the chlorinated cobalt dicarbollide (CCD) followed by precipitation of rare earths and
transuranic elements (TRU) elements as oxalates from the process raffinate is widely used in Russia for separation
of 137Cs, 90Sr, and TRU elements from the high-level radioactive waste (HLRW) solutions. Full-scale
implementation of this technology in Russia has allowed us to separate and convert into glass matrices over 50
million Curies of the long-lived radionuclides (1, 2). The principal flowsheet for this technology is shown in Fig. 1.
An inorganic, potassium-copper ferrocyanide (FS-10 type sorbent) sorption technology was used for separation of
137Cs from the acidic HLRW solutions (3). To date, this technology has been used to remove over 50 million Curies
of 137Cs from HLRW and either convert the 137Cs into a glass form or sell the 137Cs as a radiation source. The total
quantity of the long-lived radionuclides vitrified to date at the Production Association Mayak exceeds 300, 000, 000
Curie.
Organic ion exchange resins, inorganic natural and synthetic sorbents are widely used for the low-level radioactive
waste (LLRW) treatment. Those processes were extensively studied and reported. As an example, over 150000 m3
of various LLRW were decontaminated at the Production Association “Mayak”, Oziorsk, Russia using the inorganic
ferrocyanide containing sorbent.
Precipitation techniques were extensively used at the earlier stages of the radiochemical technology (4). However,
the complex problem of the liquid-solid phase separation was not solved until recently, and precipitation processes,
although simple and straightforward, were losing their significance. Clarification of solutions by means of
conventional batch filtration generated more secondary waste on account of the need for regenerating the filtering
element. Organic polymer membranes widely used in water conditioning systems appeared to be not suitable for the
purpose in most cases due to their low radiation stability.
WM’03 Conference, February 23-27, 2003, Tucson, AZ
Page - 3 -
The primary goal of our work was to develop a high performance LRW decontamination
technology using the combination of the old-fashioned precipitation processes with the state-ofthe-
art metal-ceramic “TruMem” membranes. These membranes were installed in centrifugal
filtering units operating in the continuous mode in the Membrane Sorption Reactor (MSR)
facility.
Feed solution from SNF and defense
plutonium reprocessing
Extraction
with CCD OP–10
Cs + Sr
fraction
Evaporation
Vitrification
Precipitation of oxalates
REE, Am, Cm
fraction
Evaporation
Vitrification
ILW
tank
storage
Fig. 1 Principal Flowsheet of the Separation Technology for LRW Separation
Digestion in HNO3
WM’03 Conference, February 23-27, 2003, Tucson, AZ
Page - 4 -
MEMBRANE SORPTION REACTOR DESIGN
The “TruMem” membrane is the main element of the MSR. It consists of three layers:
• support layer 200 micron thick, made of stainless steel;
• intermediate diffusion layer;
• separation layer made of the porous titanium or aluminum oxide-based ceramics (TiO2 or Al2O3) with
available pore size of 0.03 to 0.4 micron.
Separation characteristics of the “TruMem” membranes are given in the Table 1 using the LRW decontamination as
an example.
Table 1. Separation Characteristics of the “TruMem” Membranes
Membrane porosity
Radionuclide Feed Solution, Bq 0.05 micron 0.1 micron 0.4 micron
Gross β-activity 19150 13180 13420 13140
144Ce 550 < 2.3 < 2.3 < 2.3
154Eu 7030 < 2.0 < 2.0 < 2.0
241Am 1620 < 0.79 < 0.79 < 0.79
243Am 30 < 0.36 < 0.36 < 0.36
137Cs 1840 1670 1850 1870
The data in Table 1 demonstrates the ability of the TruMem membranes to retain the readily hydrolyzed
radionuclide species. Obviously, the membranes would effectively retain any fine dispersed particles as well. High
hardness of the ceramics provides for low abrasive wear.
Different layouts of the continuously operated filtration process are shown in Fig. 2. As shown in Fig. 2 the
concentration polarization, i.e. the deposit formed on the membrane surface could be removed either by high tangent
flow velocity (flat membrane unit) or by the impact of the centrifugal force and high shear stress (centrifugal
membrane unit).
Fig. 2. Different process layout with the membrane filtering elements
WM’03 Conference, February 23-27, 2003, Tucson, AZ
Page - 5 -
In our work, the simultaneous processes of the radionuclide concentrating by means of sorption on a fine dispersed
selective sorbent or co-precipitation with a special reagent additives and the continuous filtration to achieve the
liquid - solid phase separation were combined in the Membrane Sorption Reactor unit (see Fig. 3).
1 Housing 9 Separator element (or vice versa)
2 Lid 10 Peripheral collector for the 1st product
3 Inlet nipple – feed solution 11 Outlet nipple – 1st product
4 Shaft seal 12 Outlet nipple – 2nd product
5 Central shaft 13 Reaction chamber
6 Wall perforation of the shaft internal cavity 14 Inlet nipple – modifying additives – chemical
reagents and nano-sorbents
7 Membrane and separator elements – complete
set
15 Mixer shaft
8 Membrane element
Fig. 3. Schematic Drawing of the Membrane Sorption Reactor
WM’03 Conference, February 23-27, 2003, Tucson, AZ
Page - 6 -
Our MSR design provides the possibility for the sorption or co-precipitation processes to occur directly in the
reactor chamber, thus allowing for efficient reagent utilization, prompt removal of the radionuclide concentrate from
the system, and high precipitate concentrating factors.
Basic parameters of the MSR unit were determined using the results of from processing alkaline LRW to remove
137Cs by precipitation with sodium tetraphenylborate (Na-TPB) additive. The data from processing the LRW to
remove 137Cs are given in Tables 2 – 5.
Table 2. Precipitation of Cesium with NaTPB from High-Level Radioactive Waste
Solutions with Different Na Concentration
[137Cs feed] = 3.7 g/L. Precipitating agent additive in excess of Cs/NaTPB < 1/20
[Na], mole/L Precipitation factor, %
1 1.10—9 > 99.9
2 0.2 > 99.9
3 0.5 > 99.9
4 1.0 > 99.9
5 1.5 > 99.9
6 20. > 99.9
Table 3. Precipitation of Cesium with Na TPB from High-Level Radioactive Waste
Solutions with Different Na Concentration
[137Cs feed] = 30 mg/L. Precipitating agent additive in excess of Cs/NaTPB = 1/5
[Na], mole/L Precipitation factor, %
1 0.5 99.2
2 2.0 97.9
3 4.0 94.8
4 6.0 92.0
Table 4. Effect of Added TPB Quantity on the Precipitation Factor of Cesium
[Na]=2
mole/L
Cs/Na TPB ratio,
mole/mole
Cs precipitation factor,
%
1 1/5 99.94
2 1/4 99.94
3 1/3 99.95
4 1/2 99.9
5 1/1.5 99.8
WM’03 Conference, February 23-27, 2003, Tucson, AZ
Page - 7 -
Table 5. Dynamics of Cs-TPB Precipitation from Solutions of Different Composition
Using the data from Tables 2 – 5 for guidance, we selected the optimum conditions for the precipitation of cesium,
the time of contact for the feed solution and the reagent additives to determine the optimal volume of the reaction
chamber. Taking into account the filtering capacity of the “TruMem” membranes for the solutions of different
composition, we determined the surface area of the membranes to be installed in the unit to provide the throughput
needed. The desired precipitate concentrating factor is established by varying the ratio of the feed flow rate and the
concentrate removal rate. In Fig. 4, the general view of the semi-large scale MSR facility with capacity of 150 L/hr
is shown.
RESULTS OF THE MSR OPERATION
[Na] = 2 mole/L
(NaOH/NaNO3 =2/1)
[Na] = 2 mole/L
(NaOH/NaNO3 =2/1)
Precipitation time,
min
Cs precipitation factor,
%
Precipitation time, min Cs precipitation factor,
%
1 1.0 99.94 1 1.0 99.58
2 3.0 99.93 2 2.0 98.88
3 5.0 99.93 3 3.0 98.57
4 60 99.85
5 180 99.75
6 360 99.65
7 960 99.5
Fig. 4. General View of the Semi-Large Scale 150 L/hr MSR Facility
WM’03 Conference, February 23-27, 2003, Tucson, AZ
Page - 8 -
The Membrane Sorption Reactor was used for decontamination of low-level LRW of different chemical and
radiochemical composition. The results are summarized in Table 5. The data prove the high efficiency of the MSR
facility for decontamination of radioactive waste.
Table 5. LRW Decontamination With the Membrane Sorption Reactor
Firm, enterprise Volume and composition and of the
solution
Results
1 NPP “Ekoatom”
Nuclear powered submarine
decommissioning solutions
Waste volume – 50 m3
Suspensions 980 mg/L
Petroleum products 230 mg/l
137Cs – 103 Bq/L
90Sr – 103 Bq/L
60Co – 102 Bq/L
Suspensions < 3 mg/L
Petroleum products < 0.05 mg/l
137Cs < 0.3 Bq/L
90Sr < 0.3 Bq/L
60Co < 0.3 Bq/L
2 MosNPO “Radon”
Technological solutions
Waste volume – 120 m3
137Cs – 3. 103 Bq/L
90Sr – 2. 102 Bq/L
239Pu – 2.5. 103 Bq/L
Suspensions < 3 mg/L
Petroleum products < 0.05 mg/l
137Cs < 0.3 Bq/L
90Sr < 0.3 Bq/L
239Pu < 0.3 Bq/L
3 RTP “Atomflot”
Murmansk Shipping Co.
Decontamination solutions
Waste volume – 75 m3
Suspensions 10 g/L
Salt bearing 4.0 g/l
137Cs – 103 Bq/L
90Sr – 102 Bq/L
Suspensions < 3 mg/L
Hardness salts < 0.05 mg/l
137Cs < 0.3 Bq/L
90Sr < 0.3 Bq/L
4 Hydrometallurgy
plant waste
V = 120 m3/year
Radionuclides 239Pu, 241Am,
235,238U
= 103 – 104 Bq/L
pH = 7 – 14
Salt bearing 900 – 2000
mg/L
Σ 239Pu, 241Am, 235,238U
α < 1 Bq/L
5 Low-level RW of PA
“Mayak”
V = 20 m3/year
Radionuclides 137Cs, 90Sr,
FP, 241Am, 244Cm
= 102 Bq/L
= 103 Bq/L
pH = 7 – 9
Suspensions 1000 – 2000
mg/L
Σα < 0.1 Bq/L
Σβ < 1 Bq/L
Suspensions 3 mg/L
The MSR was used as the baseline technology for the facilities currently being designed for decontamination of
large LRW quantities at the Production Association “Mayak”. The design parameters for the waste type and quantity
to be treated are given in Table 6.
WM’03 Conference, February 23-27, 2003, Tucson, AZ
Page - 9 -
Table 6. MSR Facilities Under Design
Composition and volume of the feed Results
1 Hydrometallurgy plant waste
V = 120 000 m3/year
Radionuclides 239Pu, 241Am, 235,238U
= 103 – 104 Bq/L
pH = 7 – 14
Salt bearing 900 – 2000 mg/L
Σ 239Pu, 241Am, 235,238U
α < 1 Bq/L
2 Low-level RW of PA “Mayak”
V = 400 000 m3/year
Radionuclides 137Cs, 90Sr, FP, 241Am, 244Cm
= 102 Bq/L
= 103 Bq/L
pH = 7 – 9
Suspensions 1000 – 2000 mg/L
Σα < 0.1 Bq/L
Σβ < 1 Bq/L
Suspensions 3 mg/L
3 Solution after VVER fuel element digestion
600 m3/y
137Cs – up to 2 g/L
Suspensions up to 1 g/L
137Cs < 0.01 g/L
Suspensions 1-2 mg/L
POTENTIAL APPLICATIONS FOR TREATING ALKALINE RADIOACTIVE WASTE
IN THE UNITED STATES
While there are numerous potential applications of the Membrane Sorption Reactor technology for treatment of lowlevel
radioactive wastes in the United States, the following are two specific examples where this technology could
be used during treatment of wastes stored at Department of Energy facilities. The Department of Energy currently
manages approximately 206 million liters of alkaline radioactive wastes at the Hanford Site in Washington and an
additional 140 million liters of alkaline radioactive wastes at the Savannah River Site in South Carolina. The
alkaline radioactive wastes are stored in underground tanks at these sites and are comprised of salt cake, sludges,
and supernatant phases.
The Savannah River Site (SRS) currently plans to dissolve a portion of the saltcake waste and solidify a low curies
fraction in a cementitous waste form (SaltStone). Another fraction of dissolved saltcake waste may also be
solidified in SaltStone following removal of transuranic elements and 90Sr by adsorption using monosodium titanate.
The SRS currently plans to separate the monosodium titanate sorbent from the alkaline waste solution using sintered
metal, crossflow filter units. The high curie fraction of dissolved saltcake and supernatants would also be processed
to separate transuranic elements and 90Sr by adsorption using monosodium titanate, followed by 137Cs separation
using a solvent extraction process. The pretreated supernatants would then be solidified in SaltStone. Alternative
reagents (e.g., sodium permanganate) are being evaluated for removal of transuranic elements and 90Sr from the
supernatant and dissolved saltcake wastes.
SRS personnel have tested a centrifugal membrane separations unit (no reactor chamber) similar to the Membrane
Sorption Reactor and demonstrated that the centrifugal solid-liquid separation unit achieves four to six times the
throughput per area of filter media as a crossflow filter system. The Membrane Sorption Reactor combines the
chemical reaction chamber with the solid-liquid separation step, which offers the advantages of relatively high
throughput and small footprint for the radioactive equipment.
Another potential application for the MSR technology is in the treatment of sludges stored in the underground tanks
at the Hanford Site. The Department of Energy is evaluating whether some of these sludges are transuranic wastes
that could be packaged and shipped to the Waste Isolation Pilot Plant (WIPP) for disposal. The MSR could be used
to separate supernatants, remove soluble analytes and radionuclides by washing and dewater the potentially
transuranic sludges. Again, the Membrane Sorption Reactor offers the advantage of a compact processing unit with
relatively high throughput when compared to crossflow filtration or other solid-liquid separation devices.
Last Shot Hydration Drink Announced as Official Sponsor of Red River Athletic Conference • EQLB • Jun 20, 2024 2:38 PM
ATWEC Announces Major Acquisition and Lays Out Strategic Growth Plans • ATWT • Jun 20, 2024 7:09 AM
North Bay Resources Announces Composite Assays of 0.53 and 0.44 Troy Ounces per Ton Gold in Trenches B + C at Fran Gold, British Columbia • NBRI • Jun 18, 2024 9:18 AM
VAYK Assembling New Management Team for $64 Billion Domestic Market • VAYK • Jun 18, 2024 9:00 AM
Fifty 1 Labs, Inc Announces Acquisition of Drago Knives, LLC • CAFI • Jun 18, 2024 8:45 AM
Hydromer Announces Attainment of ISO 13485 Certification • HYDI • Jun 17, 2024 9:22 AM