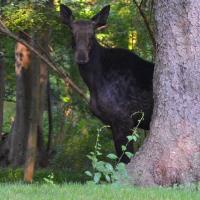
Saturday, October 29, 2011 5:57:41 PM
SILK SPINNING THE GENETICALLY MODIFIED WAY
October 27, 2011 admin No comments
Credit Randy Stewart
Despite the huge potential of genetically modified animals outside of laboratory research, commercialisation of these animals has been extremely limited. Numerous factors, including negative consumer perception, regulatory hurdles, and limitations inherent to classical GM technologies, have kept the majority of GM animal applications within the realm of academic research. However genome editing using zinc finger nucleases could help develop new markets for the future commercialisation of GM animals.
Genetic modification is commonplace throughout the life sciences sector, from fundamental research to pharmaceutical testing. GM cellular and animal models are valuable tools for the study of many chronic diseases, the testing of pharmaceutical compounds and the development of new therapeutic strategies. Genetic modification also offers great benefits in vaccine and biopharmaceutical manufacturing, which rely heavily on the use of GM organisms for biomolecule design and production. Modifying the genome of an organism or cell line allows the incorporation of target biomolecules in specific biological contexts, as well as the transfer of a gene product from a low-producing organism to one that can produce on a commercial scale. These applications have been widely accepted for many years, with countless GM organisms approved for medical manufacturing applications by drug regulators in all major countries. Despite this widespread success within the research and pharmaceutical sectors, the use of GM organisms outside of these markets has been limited.
Despite the lack of broad acceptance for most commercial applications of GM animal products, this technology has been able to gain traction in a few market sectors. The most obvious application has been the commercialisation of transgenic animals for the production of biomolecules for therapeutic use. Cattle, sheep and goats have been used for large-scale production of antibodies, steroids and hormones – most notably insulin – for many years. In 2009, GTC Biotherapeutics received US FDA approval for bioproduction of a recombinant human antithrombin. This product – ATryn – is extracted from the milk of transgenic goats, and is the first approved biopharmaceutical to be produced using genetically engineered animals. Although this is a significant breakthrough for the commercialisation of GM animals, it is still within the pharmaceutical industry, and is a natural progression of existing cell-based technologies. Of potentially greater commercial interest is the extension of genetic engineering outside of this sector, into areas such as food production, textiles and even companion animals.
GM crops have been available in many countries since the early 1990s, and numerous cash crops – including sugar beet, soybean, corn and tomatoes – have been modified to improve resistance to disease, increase the rate of growth or enhance nutritional value. However, cultivation of these transgenic crops is generally tightly regulated, particularly within the European Union, and this, together with negative public opinion, has limited the more widespread development of GM technologies.
Similar to GM crops, many of the animals currently under development are intended to confer disease resistance, an application particularly suited to the use of zinc finger nuclease (ZFN) technology. Many diseases can be treated by the targeted deletion or modification of a host gene. With ZFNs, these targets can be modified with no footprint of genetic engineering. Due to the high costs of raising livestock, another area of focus in developing commercial GM animals has been increasing the rate of growth or size of animals. Among the first GM animals likely to be launched is a fast growing salmon from AquaBounty. The AquAdvantage Salmon is designed to reach market size in half the time of a wild type salmon, reducing costs for fish farmers and limiting the environmental impact of salmon farming by avoiding the need for ocean pens.
Although genetic engineering of animals for food is primarily driven by economic pressures, GM technologies have also been used in the companion animals market. In this sector, genetic modification can be used for practical purposes – such as the creation of hypoallergenic animals or the correction of heritable congenital defects which have arisen though inbreeding – or for purely cosmetic purposes, such as GloFish. The first example of a GM pet, GloFish are fluorescent zebrafish (Danio rerio) that have had genes encoding naturally fluorescent proteins (GFP, YFP, RFP) inserted into their genome. Developed by a group at the National University of Singapore, GloFish were originally created to develop live detection systems for water pollution. They were introduced as pets in the United States in 2003 following over two years of extensive environmental research and consultation. In Europe however, the sale and possession of GloFish is prohibited by rigorous legislation concerning the use of GM technologies.
By allowing precisely targeted insertion of spider genes and concomitant removal of endogenous silkworm silk genes at the same locus, ZFN technology offers the potential for development of transgenic silkworms which will produce native spider silk at commercially viable levels
Perhaps even more interesting from a commercial perspective is the use of GM animals in the manufacture of textiles. Silkworms – actually the larval form of the silkmoth Bombyx mori – have been used for the production of silk for thousands of years, with natural silk still produced by the cultivation of silkworms today. Silkworm cocoons are unwound to create linear silk threads, then re-spun into textiles in much the same way as cotton. Although the applications of silkworm silk are numerous, due to their unique physical and chemical properties, there is also widespread interest in the silks of several other insects.
Spider silk, in particular, offers numerous possibilities within the technical textiles industry, due to its incredible tensile strength and elasticity; characteristics which have not yet been replicated in synthetic materials. Like all insect silks, spider silk fibres consist of repetitive units of protein crystals separated by less structured protein chains. The exact properties and composition of each spider silk vary with its intended function. Major Ampullate or dragline silk, for example, is relatively hydrophobic with very high tensile strength and toughness, as it is used to form the outer rim and spokes of a web. In contrast, hydrophilic capture spiral silks, which form the inner structures of the web, are sticky and highly elastic to effectively entrap prey. This high degree of variability offers enormous potential for the textiles industry, raising the possibility of tailoring the properties of silk to create advanced technical fabrics, for applications such as bulletproof vests, parachute canopies and automobile airbags; biomedical applications, including sutures and tendon and ligament repair; new fabrics, for sportswear and clothing; and even microelectronics.
Although the use of spider silks for microsutures has recently been reported, more widespread application of spider silk technologies is currently limited by the difficulty in producing silks on a commercially viable scale. This is due to the difficulties of rearing spiders in large numbers, due to their highly territorial and cannibalistic nature. As a result, the harvesting of spider silk fibres is extremely time consuming and labour intensive, with production of the only known spider silk garment – an 11 foot by 4 foot shawl made from golden orb spider silk – taking 150 people over five years to produce and costing in excess of £300,000!
To overcome these limitations, and allow future development of spider silk technologies, an alternative strategy for spider silk production is required. This makes spider silk production an obvious candidate for genetic modification, inserting spider silk genes into the genome of other silk-making insects for bioproduction. For example, random insertion of orb spider silk genes into silkworms has allowed production of hybrid spider/silkworm silk using traditional silkworm farming strategies. The resulting hybrid silk contains approximately 10% spider silk and has greater strength and durability than native silkworm silk, raising the possibility of using transgenic silkworms to produce pure spider silks.
Though straightforward in principle, the exchange of native silkworm genes for spider silk genes, alongside more widespread exploitation of genetic engineering, has been limited by the inherent restrictions of conventional GM technologies. The generalised process of modifying an organism requires several capabilities, including:
an ability to synthesise or clone the sequence of interest (if transferring genetic information);
the capacity to grow and maintain a population of the organism of interest;
access to the genome of the organism of interest;
a mechanism to select animals that have received the modification of interest.
While many different techniques exist for accomplishing each of these steps, most GM technologies offer a compromise between the efficiency of the technique and the ability to accurately and precisely target the locus of interest. Viral genomic delivery technologies effectively deliver nucleic acids to cells and organisms, but fall short on ability to target specific regions of the genome, generally only allowing random insertion of genetic material. In comparison, transposase technologies allow a greater degree of targeting, but leave unwanted traces of exogenous DNA in their wake. Other methods involve the introduction of naked DNA into the cell, which results in insertion into the genome at very low frequencies, usually at random, limiting this approach to organisms that can be economically cultivated at high densities and screened in large numbers. Simply put, most techniques for genetic manipulation are random, inefficient and leave a ‘footprint’ of foreign DNA. While this is usually tolerated in basic research, it is not acceptable for most commercial applications, and has been a major hurdle for GM animal technologies to date.
The advent of zinc finger nuclease (ZFN) technology represents a significant breakthrough for commercialisation of GM animal products, offering precisely targeted, efficient genome editing for the first time. Commercially available through Sigma Life Science under the CompoZr brand, this technique can be used to create permanent and heritable changes to an organism of interest.
This high degree of variability offers enormous potential for the textiles industry, raising the possibility of tailoring the properties of silk to create advanced technical fabrics
Credit Nir Nussbaum
ZFNs are a class of engineered DNA binding proteins that facilitate targeted editing of the genome by creating double-strand breaks at user-specified locations. These breaks stimulate the cell’s natural DNA repair mechanisms – homologous recombination (HR) and non-homologous end joining (NHEJ) – which can be exploited to achieve rapid and permanent site-specific modification of the desired genes. While HR can be used to insert foreign DNA sequences, NHEJ allows the cell’s natural processes to create precisely targeted mimics of natural mutations which leave no traces of foreign DNA. Unlike previous techniques, ZFNs offer excellent sequence specificity, binding 24 to 36 base pair target sequences to virtually eliminate off target effects, and are able to achieve modification rates exceeding 20 %, well above rates for most other technologies.
The technique is already being used to create transgenic silkworms for spider silk production. By allowing precisely targeted insertion of spider genes and concomitant removal of endogenous silkworm silk genes at the same locus, ZFN technology offers the potential for development of transgenic silkworms which will produce native spider silk at commercially viable levels.
GM technologies have revolutionised the research world and have great potential in a variety of commercial applications, but have been limited by the inherent restrictions associated with historical GM technologies. The main drawback of these technologies is their inability to accurately and efficiently target genes of interest, instead relying on random insertion of genetic material into host genomes. These limitations result in the need for extensive and costly screening to identify animals with correct transgene expression (without compromising the viability of the animal), and also result in the co-expression of both the transgene and native homologues already present.
The advent of ZFN technology signifies the beginning of an exciting new chapter in the world of genetic modification, allowing precise, targeted and efficient genome editing for the first time. Production of native spider silk using transgenic silkworms is just one example of the potential commercial applications of this innovative technology, taking us one step closer to the reality of industrial scale biomanufacturing and paving the way for an entirely new spectrum of environmentally friendly materials.
Authors:
Joseph Bedell and Brian Buntaine
Commercial Animal Technologies Group, Sigma Advanced Genetic Engineering (SAGE) Labs, Sigma Life Science
www.labnews.co.uk/features/silk-spinning-the-genetically-modified-way/
Thanks king
October 27, 2011 admin No comments
Credit Randy Stewart
Despite the huge potential of genetically modified animals outside of laboratory research, commercialisation of these animals has been extremely limited. Numerous factors, including negative consumer perception, regulatory hurdles, and limitations inherent to classical GM technologies, have kept the majority of GM animal applications within the realm of academic research. However genome editing using zinc finger nucleases could help develop new markets for the future commercialisation of GM animals.
Genetic modification is commonplace throughout the life sciences sector, from fundamental research to pharmaceutical testing. GM cellular and animal models are valuable tools for the study of many chronic diseases, the testing of pharmaceutical compounds and the development of new therapeutic strategies. Genetic modification also offers great benefits in vaccine and biopharmaceutical manufacturing, which rely heavily on the use of GM organisms for biomolecule design and production. Modifying the genome of an organism or cell line allows the incorporation of target biomolecules in specific biological contexts, as well as the transfer of a gene product from a low-producing organism to one that can produce on a commercial scale. These applications have been widely accepted for many years, with countless GM organisms approved for medical manufacturing applications by drug regulators in all major countries. Despite this widespread success within the research and pharmaceutical sectors, the use of GM organisms outside of these markets has been limited.
Despite the lack of broad acceptance for most commercial applications of GM animal products, this technology has been able to gain traction in a few market sectors. The most obvious application has been the commercialisation of transgenic animals for the production of biomolecules for therapeutic use. Cattle, sheep and goats have been used for large-scale production of antibodies, steroids and hormones – most notably insulin – for many years. In 2009, GTC Biotherapeutics received US FDA approval for bioproduction of a recombinant human antithrombin. This product – ATryn – is extracted from the milk of transgenic goats, and is the first approved biopharmaceutical to be produced using genetically engineered animals. Although this is a significant breakthrough for the commercialisation of GM animals, it is still within the pharmaceutical industry, and is a natural progression of existing cell-based technologies. Of potentially greater commercial interest is the extension of genetic engineering outside of this sector, into areas such as food production, textiles and even companion animals.
GM crops have been available in many countries since the early 1990s, and numerous cash crops – including sugar beet, soybean, corn and tomatoes – have been modified to improve resistance to disease, increase the rate of growth or enhance nutritional value. However, cultivation of these transgenic crops is generally tightly regulated, particularly within the European Union, and this, together with negative public opinion, has limited the more widespread development of GM technologies.
Similar to GM crops, many of the animals currently under development are intended to confer disease resistance, an application particularly suited to the use of zinc finger nuclease (ZFN) technology. Many diseases can be treated by the targeted deletion or modification of a host gene. With ZFNs, these targets can be modified with no footprint of genetic engineering. Due to the high costs of raising livestock, another area of focus in developing commercial GM animals has been increasing the rate of growth or size of animals. Among the first GM animals likely to be launched is a fast growing salmon from AquaBounty. The AquAdvantage Salmon is designed to reach market size in half the time of a wild type salmon, reducing costs for fish farmers and limiting the environmental impact of salmon farming by avoiding the need for ocean pens.
Although genetic engineering of animals for food is primarily driven by economic pressures, GM technologies have also been used in the companion animals market. In this sector, genetic modification can be used for practical purposes – such as the creation of hypoallergenic animals or the correction of heritable congenital defects which have arisen though inbreeding – or for purely cosmetic purposes, such as GloFish. The first example of a GM pet, GloFish are fluorescent zebrafish (Danio rerio) that have had genes encoding naturally fluorescent proteins (GFP, YFP, RFP) inserted into their genome. Developed by a group at the National University of Singapore, GloFish were originally created to develop live detection systems for water pollution. They were introduced as pets in the United States in 2003 following over two years of extensive environmental research and consultation. In Europe however, the sale and possession of GloFish is prohibited by rigorous legislation concerning the use of GM technologies.
By allowing precisely targeted insertion of spider genes and concomitant removal of endogenous silkworm silk genes at the same locus, ZFN technology offers the potential for development of transgenic silkworms which will produce native spider silk at commercially viable levels
Perhaps even more interesting from a commercial perspective is the use of GM animals in the manufacture of textiles. Silkworms – actually the larval form of the silkmoth Bombyx mori – have been used for the production of silk for thousands of years, with natural silk still produced by the cultivation of silkworms today. Silkworm cocoons are unwound to create linear silk threads, then re-spun into textiles in much the same way as cotton. Although the applications of silkworm silk are numerous, due to their unique physical and chemical properties, there is also widespread interest in the silks of several other insects.
Spider silk, in particular, offers numerous possibilities within the technical textiles industry, due to its incredible tensile strength and elasticity; characteristics which have not yet been replicated in synthetic materials. Like all insect silks, spider silk fibres consist of repetitive units of protein crystals separated by less structured protein chains. The exact properties and composition of each spider silk vary with its intended function. Major Ampullate or dragline silk, for example, is relatively hydrophobic with very high tensile strength and toughness, as it is used to form the outer rim and spokes of a web. In contrast, hydrophilic capture spiral silks, which form the inner structures of the web, are sticky and highly elastic to effectively entrap prey. This high degree of variability offers enormous potential for the textiles industry, raising the possibility of tailoring the properties of silk to create advanced technical fabrics, for applications such as bulletproof vests, parachute canopies and automobile airbags; biomedical applications, including sutures and tendon and ligament repair; new fabrics, for sportswear and clothing; and even microelectronics.
Although the use of spider silks for microsutures has recently been reported, more widespread application of spider silk technologies is currently limited by the difficulty in producing silks on a commercially viable scale. This is due to the difficulties of rearing spiders in large numbers, due to their highly territorial and cannibalistic nature. As a result, the harvesting of spider silk fibres is extremely time consuming and labour intensive, with production of the only known spider silk garment – an 11 foot by 4 foot shawl made from golden orb spider silk – taking 150 people over five years to produce and costing in excess of £300,000!
To overcome these limitations, and allow future development of spider silk technologies, an alternative strategy for spider silk production is required. This makes spider silk production an obvious candidate for genetic modification, inserting spider silk genes into the genome of other silk-making insects for bioproduction. For example, random insertion of orb spider silk genes into silkworms has allowed production of hybrid spider/silkworm silk using traditional silkworm farming strategies. The resulting hybrid silk contains approximately 10% spider silk and has greater strength and durability than native silkworm silk, raising the possibility of using transgenic silkworms to produce pure spider silks.
Though straightforward in principle, the exchange of native silkworm genes for spider silk genes, alongside more widespread exploitation of genetic engineering, has been limited by the inherent restrictions of conventional GM technologies. The generalised process of modifying an organism requires several capabilities, including:
an ability to synthesise or clone the sequence of interest (if transferring genetic information);
the capacity to grow and maintain a population of the organism of interest;
access to the genome of the organism of interest;
a mechanism to select animals that have received the modification of interest.
While many different techniques exist for accomplishing each of these steps, most GM technologies offer a compromise between the efficiency of the technique and the ability to accurately and precisely target the locus of interest. Viral genomic delivery technologies effectively deliver nucleic acids to cells and organisms, but fall short on ability to target specific regions of the genome, generally only allowing random insertion of genetic material. In comparison, transposase technologies allow a greater degree of targeting, but leave unwanted traces of exogenous DNA in their wake. Other methods involve the introduction of naked DNA into the cell, which results in insertion into the genome at very low frequencies, usually at random, limiting this approach to organisms that can be economically cultivated at high densities and screened in large numbers. Simply put, most techniques for genetic manipulation are random, inefficient and leave a ‘footprint’ of foreign DNA. While this is usually tolerated in basic research, it is not acceptable for most commercial applications, and has been a major hurdle for GM animal technologies to date.
The advent of zinc finger nuclease (ZFN) technology represents a significant breakthrough for commercialisation of GM animal products, offering precisely targeted, efficient genome editing for the first time. Commercially available through Sigma Life Science under the CompoZr brand, this technique can be used to create permanent and heritable changes to an organism of interest.
This high degree of variability offers enormous potential for the textiles industry, raising the possibility of tailoring the properties of silk to create advanced technical fabrics
Credit Nir Nussbaum
ZFNs are a class of engineered DNA binding proteins that facilitate targeted editing of the genome by creating double-strand breaks at user-specified locations. These breaks stimulate the cell’s natural DNA repair mechanisms – homologous recombination (HR) and non-homologous end joining (NHEJ) – which can be exploited to achieve rapid and permanent site-specific modification of the desired genes. While HR can be used to insert foreign DNA sequences, NHEJ allows the cell’s natural processes to create precisely targeted mimics of natural mutations which leave no traces of foreign DNA. Unlike previous techniques, ZFNs offer excellent sequence specificity, binding 24 to 36 base pair target sequences to virtually eliminate off target effects, and are able to achieve modification rates exceeding 20 %, well above rates for most other technologies.
The technique is already being used to create transgenic silkworms for spider silk production. By allowing precisely targeted insertion of spider genes and concomitant removal of endogenous silkworm silk genes at the same locus, ZFN technology offers the potential for development of transgenic silkworms which will produce native spider silk at commercially viable levels.
GM technologies have revolutionised the research world and have great potential in a variety of commercial applications, but have been limited by the inherent restrictions associated with historical GM technologies. The main drawback of these technologies is their inability to accurately and efficiently target genes of interest, instead relying on random insertion of genetic material into host genomes. These limitations result in the need for extensive and costly screening to identify animals with correct transgene expression (without compromising the viability of the animal), and also result in the co-expression of both the transgene and native homologues already present.
The advent of ZFN technology signifies the beginning of an exciting new chapter in the world of genetic modification, allowing precise, targeted and efficient genome editing for the first time. Production of native spider silk using transgenic silkworms is just one example of the potential commercial applications of this innovative technology, taking us one step closer to the reality of industrial scale biomanufacturing and paving the way for an entirely new spectrum of environmentally friendly materials.
Authors:
Joseph Bedell and Brian Buntaine
Commercial Animal Technologies Group, Sigma Advanced Genetic Engineering (SAGE) Labs, Sigma Life Science
www.labnews.co.uk/features/silk-spinning-the-genetically-modified-way/
Thanks king
Join the InvestorsHub Community
Register for free to join our community of investors and share your ideas. You will also get access to streaming quotes, interactive charts, trades, portfolio, live options flow and more tools.